INTRODUCTION
Microsatellite marker genotyping has been a widely and successfully used technique in several areas of plant genetic research and development, including genetic mapping, population genetics, QTL analysis, forensics, and diagnostics (Powell et al., 1996; Schlotterer, 2004; Varsheney et al., 2005). Although several methods are used for resolving microsatellite alleles, the current quality standard is based on automated systems using fluorescently labeled polymerase chain reaction (PCR) fragments that allow very precise allele calling, a particularly important aspect in plant population analysis to avoid genotyping errors (Schwengel et al., 1994; Bonin et al., 2004; Hoffman and Amos, 2005). In this method, one of the primers used in the PCR is 5’ end labeled with a fluorescent dye. However, the cost of synthesizing fluorescently labeled primers is a limiting factor for many labs, as labeled primers cost between five and ten times more than unlabeled ones. Furthermore, in plant genetic analysis, such primers are frequently only needed to genotype just a few tens or hundreds of samples, and are then stored for variable amounts of time during which the fluorescence can quickly decay. This is particularly common in laboratories that work with several plant species addressing community-wide population genetics and conservation questions.
To reduce the costs of genotyping with fluorescently labeled microsatellites, Oetting et al. (1995) proposed a PCR strategy, called multiplexing with tailed primers, which employs a forward primer with a 19-bp extension at its 5’ end, identical to the sequence of an M13 sequencing primer, a regular reverse primer and a third universal fluorescent labeled M13 primer. In this three-primer strategy, detection is thus based on the addition of a sequence that has no homology with the target genome, to the 5’ end of the forward primer of the microsatellite marker. This primer “tail” provides a complementary sequence to the universal fluorescent primer starting from the third PCR cycle, generating a fluorescent product that can be detected on an automatic DNA sequencer. Therefore, instead of synthesizing one specific labeled forward primer for each microsatellite marker, only one universal labeled primer is needed. Various groups later proposed fundamentally the same strategy. Neilan et al. (1997) described the same method; however, they proposed that the labeled primer could be designed to have a unique sequence, exhibiting insignificant homology to any sequence found in DNA databases. Schuelke (2000) used the same strategy to type a locus on the human X chromosome using an M13 tailed primer and 6-FAM fluorescent labeling. Guo and Milewicz (2003) used two universal labeled primers with 6-FAM and HEX dyes, respectively, for typing human DNA samples. Although most of these studies used the M13 sequence as a tail, any other sequence that does not have a complementary sequence in the target genome under study could potentially be used, as pointed out by Neilan et al. (1997). Furthermore, although cost-efficient, the M13 tailing method limits multiplexing to marker loci whose alleles migrate to non-overlapping size ranges.
Based on the well-known concept of a universal tailed primer, our objective was to test the simultaneous use of three different universal tailed primers, one for each fluorescence detection. Such a method would permit the same level of marker multiplexing and accuracy in plant microsatellite genotyping attained in regular microsatellite fluorescent detection assays, while at the same time allowing significant cost reduction. This method was tested for the simultaneous detection of Eucalyptus microsatellites in a genetic mapping experiment.
MATERIAL AND METHODS
Universal primer selection
Three primers with 20 bases used for human genotyping without significant complementarity to any plant sequence, verified by BLASTn against Genbank, were selected to be used as universal tails (Table 1). These primers were the forward primer of microsatellite DYS437 labeled with 6-FAM, the forward primer of microsatellite D8S1132 labeled with HEX, and the forward primer of microsatellite D12S1090 labeled with NED. Each of the forward primers of a set of Eucalyptus microsatellite markers (Brondani et al., 2002) was synthesized with an added 5’ tail. The tail corresponded exactly to the sequence and orientation of the universal primer to be used (Table 1). The combination of which tail to add to which marker locus was such that loci with non-overlapping allele size ranges received the same tail, so that up to six marker loci could be multiplexed, although a larger number could be combined depending on the size ranges of the target loci.
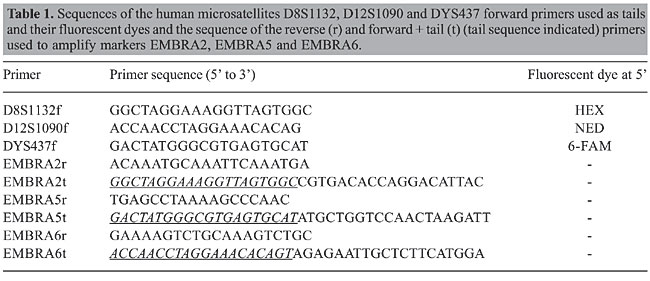
PCR conditions
The PCR reaction was prepared in a 20-µL volume, containing 1X PCR buffer (10 mM Tris-HCl, pH 8.3, 50 mM KCl), 1.5 mM MgCl2, 0.19 µM dNTP, 0.24 µg/µL BSA (New England Biolabs), 1.0 U Taq polymerase (Phoneutria Biotecnologia, Brazil) and 6.0 ng eucalypt DNA extracted from expanded leaves, as described earlier (Grattapaglia and Sederoff, 1994). To the PCR mix for each microsatellite marker, 0.1 µM of each one of the three primers (i.e., forward tailed primer, reverse primer and labeled universal primer) were added. PCR amplification was conducted as follows: 94°C (5 min), then 30 cycles at 94°C (1 min) /57°C (1 min) /72°C (1 min), and a final extension at 72°C for 30 min. The PCR reactions were carried out separately for each microsatellite, and mixtures of PCR products of three or more different markers with different dyes were made for simultaneous detection of the amplified alleles. Subsequently, 1.0 µL of the PCR product mixture was added to 10 µL formamide and 1.0 µL fragment size standard labeled with ROX (Brondani and Grattapaglia, 2001) and run on an ABI 3100 DNA analyzer (Applied Biosystems). The data were collected automatically by the detection of the different fluorescences and analyzed by GeneScan/Genotyper softwares (Applied Biosystems).
Verification of genotyping quality
Two tests were carried out to verify the correct interpretation of the microsatellite genotypes. The first aimed to check the efficiency and the expected difference in base pairs between the conventional and the multiple-tailed primer method. A set of 12 Eucalyptus plants were genotyped for markers EMBRA2, EMBRA5 and EMBRA6, using the standard labeled primers labeled with the fluorochromes HEX, 6-FAM and NED, respectively, and the multiple-tailed primer method. The second test consisted of an inheritance and linkage analysis of four markers that belong to the same linkage group in Eucalyptus (Brondani et al., 2002; Missiaggia, 2005). These markers were EMBRA60, EMBRA164, EMBRA228, and EMBRA65.
RESULTS AND DISCUSSION
We extended the concept and methodology introduced by Oetting et al. (1995) by proposing the simultaneous use of three different universal tailed primers to allow significant time and cost savings in medium throughput microsatellite genotyping experiments of plant species. Three forward primer sequences of human microsatellite markers were used as tails to genotype Eucalyptus DNA samples. No particular rule was used to choose these primers, besides their immediate availability and proven reliability in PCR. The robustness of the method was tested for the simultaneous detection and genetic analysis of Eucalyptus microsatellites in a genetic mapping experiment. In contrast to other studies that used a single dye, our method uses three different fluorescent dyes: HEX, NED and 6-FAM. It therefore allows the same level of multiplexing attained in regular microsatellite fluorescent detection assays.
Examination of genotyping quality revealed, as expected, that the observed difference in allele sizes between the alleles amplified using a regular fluorescence labeled primer and the multiple-tailed primers was 20 bp at all three loci in all individuals. This result demonstrates that the amplifications took place in the same and correct manner with the multiple-tailed primer method (Figure 1). This same result was obtained for all 45 microsatellites that were genotyped using this method in a set of 12 Eucalyptus plants. The inheritance and linkage analysis test also yielded the expected results, with all four markers mapping as expected when compared to the linkage mapping information previously generated with regular fluorescent loci.
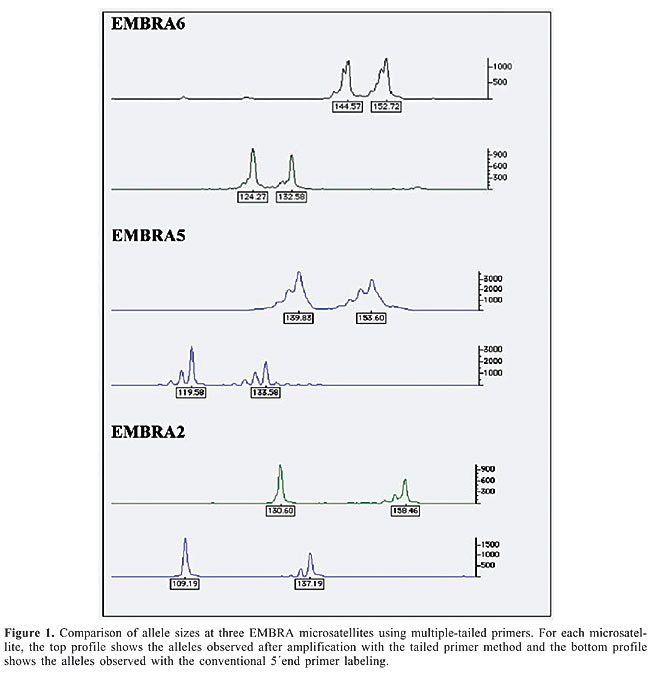
The analysis of the microsatellites amplified by the multiple-tailed primer method was assessed by multiplex loading, i.e., each locus was amplified separately and combined to be electroinjected into the same capillary. The ability to multiplex amplify several microsatellite markers in the same PCR reaction was not our objective here. Such an optimization is in fact a species and microsatellite marker specific step that has to be performed irrespective of whether one uses or not the multiple-tailed primer method described. Some preliminary multiplexing tests carried out (data not shown) indicated, however, that for up to three markers, multiplexing with multiple-tailed primers is no more difficult than multiplexing in the conventional fluorescent detection method with specific end-labeled forward primers. Multiplex PCR primer design and optimization is still mostly empirical and considerably more challenging than designing single locus PCR primer pairs because multiple primer annealing events need to occur under the same annealing conditions without interfering with one another. Systematic protocols for designing and testing multiplexes have been recently proposed (Schoske et al., 2003; Butler, 2005), together with softwares to test for multiple primer complementarity (e.g., Vallone and Butler, 2004) and commercially available kits of optimized reagent mixes for multiplex PCR. The adoption of such protocols and the flexibility of testing different human microsatellite primers to be used as tails, given the vast availability of human microsatellites, should allow the effective development of larger multiplex sets for any plant species based on this multiple-tailed primer method.
In principle, primer sequences of any human microsatellite marker could be used as tails with the same efficiency as the ones that we used. Randomly generated sequences with no homology to any plant sequence could also be equally effective. However, the use of known microsatellite primer sequences from humans to amplify plant DNA with the multiple primer method has several advantages: 1) microsatellite markers derived from genomic non-coding DNA clones are highly selected to be species specific and therefore with little if any probability of cross amplification of plant DNA; 2) microsatellite primers are typically selected and tested to yield robust PCR products of easy interpretation, and 3) several fluorescent labeled human microsatellite primers are usually available in neighboring human genetic laboratories on campus and can often be freely obtained or alternatively purchased as stock products at very competitive prices when compared to custom made ones. Furthermore, the multiple-tailed primer method could be applied as efficiently in an inverse format, i.e., using plant-specific microsatellite primer sequences to carry out amplification of human or animal DNA. Considering that microsatellites are rarely transferable across distant taxa, it should also be possible to use, for example, maize microsatellite primer sequences as tails when amplifying a dicot microsatellite from soybean, for example.
Besides the increased quality of the genotypic data provided by the fluorescent detection method when compared to colorimetric ones such as silver nitrate, the savings achieved with this method become greater with increasing numbers of microsatellite markers. Laboratories studying several different species would benefit the most from the common use of multiple-tailed primers.
For example, at a cost of US$0.50/base and US$80.00/fluorochrome end labeling of the forward primer, the cost of primers for a microsatellite amplified with two 20-base primers would be US$100.00. The cost of primers for fluorescence-based detection of ten microsatellite markers would be US$1,000.00. On the other hand, with the multiple-tailed primer method the cost of the unlabeled primers per microsatellite marker would be US$30.00, i.e., 40 bases for the forward tailed primer and 20 bases for the reverse, a total of 60 bases at 0.50/base. For fluorescence detection, only three universal labeled primers are needed, each with 20 bases. The universal primers would be used for every microsatellite marker, and thus would have to be synthesized on a larger scale, the cost per base going up from US$0.50 to 1.00/base. The cost of synthesis of the three universal primers would be US$60.00, plus US$240.00 for the three fluorochrome end labeling, yielding a total of US$300.00. The cost of primers for fluorescence-based detection of 10 microsatellite markers would total US$600.00, an economy of 40% when compared to the standard method.
For the analysis of a larger set of microsatellites, the cost of the standard method increases linearly. For example for a 200-marker project, it would be 200 x 100 = US$20,000. With the multiple-tailed primer method the cost increase is not linear, but rather the cost decreases on a per marker basis as the number of markers increases. For example for a set of 200 markers the cost would be 200 x 30.00 = US$6000.00 for the unlabeled primers and the same US$300.00 for the labeled universal primers, totaling US$6,300.00, a saving of 68.5% when compared to the standard method.
In conclusion, by using multiple-tailed primers derived from human microsatellites with no homology with the target plant species, one can obtain reliable and cost-effective genotyping in a fluorescent multiplex system. The cost reduction becomes especially sizeable as the number of markers to be analyzed increases, because the cost of the three universal labeled primers is diluted. This approach has been applied to genotype a number of tropical forest tree species in our lab, increasing the speed and quality of data generation when compared to staining detection methods, at a fraction of the cost of conventional fluorescent microsatellite detection.
ACKNOWLEDGMENTS
Research supported by the Brazilian Ministry of Science and Technology - FINEP through the competitive grant GENOLYPTUS - “Brazilian Network of Eucalyptus Genome Research” to D. Grattapaglia and collaborators, and the Brazilian National Research Council - CNPq with a Ph.D fellowship to A. Missiaggia and a research fellowship to D. Grattapaglia.
REFERENCES
Bonin A, Bellemain E, Bronken EP, Pompanon F et al. (2004). How to track and assess genotyping errors in population genetics studies. Mol. Ecol. 13: 3261-3273.
Brondani RP and Grattapaglia D (2001). Cost-effective method to synthesize a fluorescent internal DNA standard for automated fragment sizing. Biotechniques 31: 793-800.
Brondani RP, Brondani C and Grattapaglia D (2002). Towards a genus-wide reference linkage map for Eucalyptus based exclusively on highly informative microsatellite markers. Mol. Genet. Genomics 267: 338-347.
Butler JM (2005). Constructing STR multiplex assays. Methods Mol. Biol. 297: 53-66.
Grattapaglia D and Sederoff R (1994). Genetic linkage maps of Eucalyptus grandis and Eucalyptus urophylla using a pseudo-testcross: mapping strategy and RAPD markers. Genetics 137: 1121-1137.
Guo DC and Milewicz DM (2003). Methodology for using a universal primer to label amplified DNA segments for molecular analysis. Biotechnol. Lett. 25: 2079-2083.
Hoffman JI and Amos W (2005). Microsatellite genotyping errors: detection approaches, common sources and consequences for paternal exclusion. Mol. Ecol. 14: 599-612.
Missiaggia AA (2005). Genetic mapping of QTL for wood quality and early flowering and allele specific gene expression studies of lignification genes in Eucalyptus. Ph.D. thesis, Universidade de São Paulo, USP, São Paulo, SP, Brazil.
Neilan BA, Wilton AN and Jacobs D (1997). A universal procedure for primer labelling of amplicons. Nucleic Acids Res. 25: 2938-2939.
Oetting WS, Lee HK, Flanders DJ, Wiesner GL et al. (1995). Linkage analysis with multiplexed short tandem repeat polymorphisms using infrared fluorescence and M13 tailed primers. Genomics 30: 450-458.
Powell W, Machray GC and Provan J (1996). Polymorphism revealed by simple sequence repeats. Trends Plant Sci. 1: 215-222.
Schlotterer C (2004). The evolution of molecular markers - just a matter of fashion? Nat. Rev. Genet. 5: 63-69.
Schoske R, Vallone PM, Ruitberg CM and Butler JM (2003). Multiplex PCR design strategy used for the simultaneous amplification of 10 Y chromosome short tandem repeat (STR) loci. Anal. Bioanal. Chem. 375: 333-343.
Schuelke M (2000). An economic method for the fluorescent labeling of PCR fragments. Nat. Biotechnol. 18: 233-234.
Schwengel DA, Jedlicka AE, Nanthakumar EJ, Weber JL et al. (1994). Comparison of fluorescence-based semi-automated genotyping of multiple microsatellite loci with autoradiographic techniques. Genomics 22: 46-54.
Vallone PM and Butler JM (2004). AutoDimer: a screening tool for primer-dimer and hairpin structures. Biotechniques 37: 226-231.
Varshney RK, Graner A and Sorrells ME (2005). Genic microsatellite markers in plants: features and applications. Trends Biotechnol. 23: 48-55.