|
- Review
- Female remating, sperm competition and sexual selection in Drosophila
- Shree Ram Singh1, Bashisth N. Singh1 and Hugo F. Hoenigsberg2
- 1Genetics Laboratory, Department of Zoology, Banaras Hindu University, Varanasi 221 005, India
2Departmento de Genetica, Universidad Manuela Beltran, Bogota, DC, Colombia
- Corresponding author: B.N. Singh/H.F. Hoenigsberg
- E-mail: [email protected]/[email protected]
- Genet. Mol. Res. 1 (3): 178-215 (2002)
- Received April 29, 2002
- Published August 5, 2002
ABSTRACT. Female remating is fundamental to evolutionary biology as it determines the pattern of sexual selection and sexual conflict. Remating in females is an important component of Drosophila mating systems because it affects sperm usage patterns and sexual selection. Remating is common in females of many species of Drosophila in both natural and laboratory populations. It has been reported in many insect species and also in vertebrates. Female remating is a prerequisite for sperm competition between males, and the consequences of this competition, such as sperm precedence or sperm displacement, have been reported for many species of Drosophila. Female remating is dependent on the amount of sperm stored, the male seminal fluid components, nutrition, the quantity of eggs laid, experimental design and density of flies in laboratory. Remating by a female is an insurance against male sterility and sub-fertility and increases genetic heterogeneity of female offspring. Remating gives greater female productivity in many species of Drosophila. We examined female remating with respect to sperm competition and sexual selection in Drosophila and addressed the possible benefits for females. We also reviewed the role of accessory gland fluid in remating, costs associated with remating, the genetic basis of female remating and some possible mechanisms of sperm competition in the light of last male sperm priority and paternity assurance in Drosophila and other insects. We also suggest future areas of research.
Key words: Drosophila, Drosophila mating system, Remating, Sperm competition, Sexual selection
INTRODUCTION
Mating is the most important and fundamental process for animals to select the best partner and to produce progeny. Darwin's theory of sexual selection can help model the basic patterns of choosing a mate. According to Darwin (1859), sexual selection is the struggle for existence in the face of other organic beings or external conditions. This means the struggle of one sex (usually the male), trying to perpetuate his genes by winning the acceptance of and mating with the female. The result of failure is normally not death, but few or no offspring. The refused male's genes are not carried on and thus gene diversity in the alleles diminishes. In the opinion of Darwin (1871), sexual selection is actually nonrandom differential reproductive success that results from competition for access to mates. However, the optimal strategy for males may not be to mate with as many females as possible, but rather to fertilize as many eggs as possible. The influence of sexual selection can only affect one sex directly (usually the male) and the other indirectly (usually the female). It also requires that there be an uneven ratio of male to female organisms so as to keep the competition intense.
In other words, sexual selection arises from the differential mating success of individuals of one sex. There are two mechanisms that may lead to differential mating success: competition among individuals of the "chosen" sex for access to individuals of the "choosy" sex or "power to conquer other males in battle", usually male-male competition (intra-sexual selection (Darwin, 1871; Parker, 1970)) and choice of attractive partners by individuals of the "choosy" sex or "the power to charm", usually female choice (inter-sexual selection (Eberhard, 1996)). According to Møller (1994) sexual selection is a continuous process that takes place at a number of different stages in reproduction, from the actual process of mate acquisition through copulation, subsequent fertilization, differential abortion and infanticide and differential investment in offspring.
Mating by animals is an important component of sexual behavior (Hoenigsberg, 1960) and transfer of sperm to females is the primary function of mating in sexually reproducing animals. Since each mating provides an opportunity to produce offspring, males can generally increase their fitness by mating with many females and by high male reproductive success (Sing and Sing, 2000). However, females increase their reproductive success by increasing the number of viable eggs produced. This basic asymmetry between the sexes suggests that male fitness increases monotonically with increased mating rate, while single or a few matings are sufficient for females to maximize their reproductive success. However, females of a majority of animal species mate multiply, most often with different males (polyandry-multiple mating or remating) but also with same male (repeated mating) (Arnquist and Nilson, 2000).
Female remating is an important component of Drosophila mating systems because females store the large quantity of sperm after mating in paired spherical spermathecae and single elongate tubular seminal receptacles (Pitnick et al., 1999; Tram and Wolfner, 1999; Neubaum and Wolfner, 1999), and utilize them to fertilize eggs as they are laid (Wolfner, 1997). Once a virgin female Drosophila has mated, she is usually unwilling to accept another male for some time. The female is usually the discriminating or limiting partner in the mating act, i.e. she actively accepts or rejects a courting male. The length of this refractory period varies between species and in different strains of the same species (Manning, 1962). But Drosophila females may also mate again before the sperm from a previous mating have been exhausted (Lefevre and Jonsson, 1962). After mating, behavioral and physiological changes are seen in Drosophila females, which include: a decrease in attractiveness to males (Tram and Wolfner, 1998), less movement in response to males (Tompkins et al., 1982), decreased receptivity to further mating (Fuyama, 1995; Ringo, 1996), increased oogenesis, ovulation and oviposition rates (Heifetz et al., 2001), storage and utilization of sperm (Gromko et al., 1984), and decreased life span (Chapman et al., 1995; Sgro and Partridge, 1999). These behavioral and physiological alterations in females after mating have both short- and long-term effects. The short-term effect, called the "copulation effect" in Drosophila, is due to seminal fluid components (proteins) transferred during mating by males, causing an initial decrease in receptivity (Wolfner, 1997; Neubaum and Wolfner, 1999), thus maximizing sperm usage and minimizing the sperm competition. Long-term suppression of female remating in Drosophila has been indirectly linked to sperm load and is termed the "sperm effect" (Manning, 1962; Gromko et al., 1984; Scott, 1987). Copulation and sperm effects are not necessarily mutually exclusive and could both be present during the first day after mating. However, in a normal mating both sperm and seminal fluid is transferred, so copulation effects would usually obscure any sperm effects within a day. Tompkins and Hall (1983) reported that the central nervous system (CNS) proximately controls receptivity of D. melanogaster females. Mechanical stimulation of the CNS by sperm movement, detected by innervations of the sperm storage organs, has been suggested to mediate long-term inhibition of female remating (Gromko et al., 1984).
We examined the results of remating studies in various species of Drosophila, as well as in other insects and vertebrates. We also looked at the consequences of sperm competition on female remating, such as sperm displacement and sperm precedence, and the possible benefits of female remating and sperm competition mechanisms. We pose the following questions: i) Why do females engage in copulation with more than one male? ii) Why do inseminated females mate again, often long before the supply of sperm they carry is exhausted? iii) Is there a selective advantage to females who mate repeatedly? iv) How does sperm competition influence the intensity of sexual selection? v) Which factors determine a male's success in sperm competition? vi) To what extent and how can females control paternity and sex in their offspring? vii) Is there any cost when a female remates? and viii) Does female remating have a genetic basis? These questions are important for us to determine how evolutionary forces shape species morphology and behavior.
FEMALE REMATING AND SPERM COMPETITION IN DROSOPHILA
Reproductive capacity is a good index for fitness in organisms that go through repeated cycles of rapid population growth. In such organisms any feature of the reproductive biology that increases the reproductive rate will be favored by natural selection. Remating and sperm storage are specific features that can play important roles in determining female fecundity and male mating success, and hence fitness (Levine et al., 1980). Female remating is common in many species of Drosophila under both natural and laboratory conditions (Richmond and Powell, 1970; Milkman and Zeitler, 1974; Ikeda, 1974; Anderson, 1974; Richmond and Ehrman, 1974; Richmond, 1976; Stalker, 1976; Prout and Bundgaard, 1977; Cobbs, 1977; Craddock and Johnson, 1978; Gromko and Pyle, 1978; Gromko et al., 1980; Loukas et al., 1981; Griffiths et al., 1982; Markow and Ankey, 1984; Markow, 1982, 1985; Turner, 1986; Marks et al., 1988; Bressac et al., 1991; Aspi, 1992; Aspi and Lankinen, 1992; Schwartz and Boake, 1992; Etges and Heed, 1992; Pitnick and Markow, 1994; Joly et al., 1995; Price, 1997; McRobert et al., 1997; Harshman and Clark, 1998; Snook, 1998; Hirai and Kimura, 1999; Singh and Singh, 1999; Bundgaard and Barker, 2000; Mery and Joly, 2002, also see Table 1).
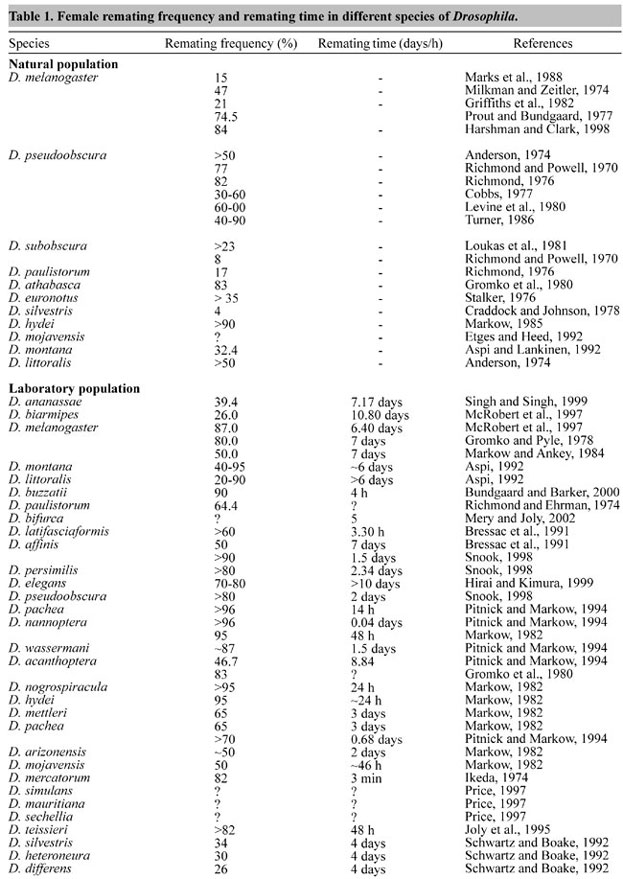
It has also been proven with DNA marker technology (molecular genetic techniques) that females frequently mate with more than one male in a wide array of species (Eberhard, 1996; Birkead and Møller, 1998; Imhoff et al., 1998). Female remating is reported in many insect (Simmons and Siva-Jothy, 1998), fish (Garant et al., 2001), reptile (Pearse and Avise, 2001), bird (Birkead and Møller, 1998), and mammal (Baker and Bellis, 1993) species. The phenomenon of female remating has received considerable attention because it is associated with sperm usage patterns and sexual selection (Parker, 1970; Smith, 1984; Pitnick and Markow, 1994; Harshman and Clark, 1998; Danielsson, 1998; Civetta, 1999). Parker (1970) used the term "sperm competition" and defined sperm competition as "competition within a female between the sperm from two or more males for the fertilization of the ova". He further defined sperm competition as "sperm competition games" to stress that they are evolutionary games between rival males, whose ejaculates compete for the fertilization of the ova. Sperm competition should therefore exert a strong evolutionary pressure on characteristics that allow males to pre-empt the sperm stored by females from previous matings, and/or to avoid sperm pre-emption by males that encounter the female after mating.
Among animals there is considerable variation in the extent to which females remate (Halliday and Arnold, 1987). This diversity can be seen among the members of the genus Drosophila, where D. pachea females have been reported to remate several times in a day (Pitnick et al., 1991), while in D. subobscura, Maynard-Smith (1956) has shown that females rarely mate a second time. However, Loukas et al. (1981) reported that >23% females were inseminated at least twice in D. subobscura after analyzing single female offspring using two highly polymorphic enzyme systems (Est-5 and Est-9). Milkman and Zeitler (1974) observed a 47% remating frequency in caged and natural populations of D. melanogaster, on the basis of the genotypes at three loci (a-GPDH, MDH and ADH). D. melanogaster females, provided with males every morning, usually will not remate for about 5 to 7 days according to Pyle and Gromko (1978). Prout and Bundgaard (1977) found that 74.5% of the females mated twice in D. melanogaster when genetic marker stocks were used for confirmation. Dobzhansky and Pavlovsky (1967) first reported female remating in natural and laboratory populations of D. pseudoobscura. Later, other investigators found variations in the frequency of female remating in this species, ranging from 40 to >90% in different populations (Anderson, 1974; Cobbs, 1977; Levine et al., 1980). Craddock and Johnson (1978) studied mating in natural populations of D. silvestris with inversion markers and found 4% female remating; they proposed that multiple insemination is a limited phenomenon in D. silvestris. Markow (1985) observed >90% remating in D. hydei and also observed that D. hydei females would remate as often as four times in one morning. D. mojavensis females remate daily, exhibit a copulatory plug and have been shown to obtain a contribution from the male ejaculate (Markow and Ankey, 1984). Richmond and Powell (1970) reported that 8% of female D. paulistorum in wild populations mated twice. However, Richmond and Ehrman (1974) calculated 64.4% female remating in D. paulistorum in culture vials and in population cages on the basis of tetrazolium oxidase genotypes, in two experiments and white-eyed, in a third. Aspi (1992) studied the incidence, timing and adaptive significance of multiple mating in females of D. littoralis and D. montana under laboratory conditions. In both species, all the females were willing to remate within 6 days, although D. littoralis females showed a tendency to remate later than the D. montana. He also observed that about 40% of D. montana females remated four hours after the initial mating, whereas only small proportion (<20%) did so in D. littoralis. Barbadilla et al. (1991) reported 90% remating in the cactophilic species, D. buzzatii. Remating in the field has also been reported as >35% for D. eronotus (Stalker, 1976) and 83% for D. athabasca (Gromko et al., 1980). Imhoff et al. (1998) tested for female remating in wild populations of D. melanogaster with highly polymorphic microsatellite and identified the genotypes of four to six different males in the offspring of each female. They found a high frequency of female remating, as females were mated with more than two males. Singh and Singh (1999) reported 21 to 56% female remating frequency in different laboratory strains of D. ananassae.
Which experimental design, periodic interaction or continuous confinement, is most similar to what occurs in natural populations? There is evidence that supports the idea that periodic interaction is a better model for remating in nature: i) Hardeland (1972) reported that courtship activity in Drosophila was circadian and was highest in the last few hours before the light portion of the light-dark cycle, ii) Stalker (1976) presented a sperm load hypothesis for wild-caught females, and iii) Pyle and Gromko (1978) showed an adaptive advantage for female remating. According to these studies, a periodic interaction model has a better fit than a continuous confinement model. The receptivity of female to remating depends upon the number of sperm in storage, which increases the relative reproductive success of females and of males whose sperm are in storage. In female remating studies two experimental designs have been most widely used by various investigators, these are continuous confinement and periodic confinement; female remating frequency has been found to vary considerably in the different species (Maynard-Smith, 1956; Richmond and Ehrman, 1974; Prout and Bundgaard, 1977; Gromko and Pyle, 1978; Pyle and Gromko, 1978; Loukas et al., 1981; Markow and Ankey, 1984; Turner and Anderson, 1984; Markow, 1985; Barbadilla et al., 1991; Aspi, 1992; McRobert et al., 1997; Singh and Singh, 1999). In female remating studies the 2-h periodic confinement technique has been used by Gromko and Pyle (1978), Newport and Gromko (1984), Letsinger and Gromko (1985), Trevitt et al. (1988) and Service and Vossbrink (1996) in D. melanogaster, McRobert et al. (1997) in D. biarmipes and D. melanogaster and Singh and Singh (1999) in D. ananassae. In D. melanogaster, it was found that about 80% of the females remate when remating was observed for 12 days (Gromko and Pyle, 1978). McRobert et al. (1997) studied remating in females of D. melanogaster and D. biarmipes during 2-h periodic confinement for 14 days and compared the post-copulatory behavior of D. biarmipes and D. melanogaster females. Females of both species were shown to undergo a series of behavioral changes following mating, including a significant reduction in both sexual attractiveness and receptivity. However, while both attractiveness and receptivity return to "virgin like" levels within a few days in D. melanogaster, D. biarmipes females, which regained their sexual attractiveness within a few days, remained unreceptive to copulation for at least two weeks. They also tested remating frequency in both species and found that about 26% of D. biarmipes females mated at least twice and that the mean remating latency was 10.8 days. In contrast, in D. melanogaster, 87% of the females remated and the remating latency was 6.5 days. In D. ananassae, a 2-h periodic confinement technique was used and remating was observed for 12 days; the average female remating frequency was 39.4% and the mean remating latency was 7.17 days (Singh and Singh, 1999). Joly et al. (1991) and Joly and Lachaise (1993) studied female remating and compared the duration of copulation in first and second matings in two geographic strains of D. teisseri, using a periodic confinement technique (8 h), and scored remating after 24, 48 and 72 h. They found a significant strain variation in female remating frequency. They also compared the duration of copulation in first and second matings and found a longer duration of copulation during the second mating (Joly et al., 1991). Interestingly, the strains of D. teissieri differed in sperm morphology. Based on their findings with D. teissieri, Joly et al. (1991) proposed that the stable coexistence of two fertile sperm morphs (short and long) in one ejaculate is a mixed strategy, which evolved via sperm competition, possibly in response to facultative female polyandry. Bressac et al. (1991) found a high recurrence of polyandry in D. littoralis and D. latifasciaeformis (up to three mates within eight hours) and a low recurrence of polyandry in D. affinis (females had only two rematings every seven days). They also measured the duration of copulation in first as well as in subsequent matings. Interestingly, there was no significant difference in the duration of copulation in the different matings in D. littoralis and D. latifasciaeformis. However, there was a significant decrease in the duration of copulation from the first to the second mating in D. affinis, which has low recurrence polyandry. D. ananassae females were also found to have a significantly shorter duration of copulation during the second mating (remating) (Singh and Singh, 1999). Singh and Singh, 1999 also concluded that there is significant variation among species and that species with a low frequency of remating takes less time for copulation in second matings. However, recently Bundgaard and Barker (2000) found a shorter duration of copulation in the second mating when compared to the first mating in D. buzzatii females, though this species is the fastest remating group among Drosophila species. Long copulation is an adaptation for males, which can reduce the risk of sperm competition with future ejaculates by producing a mating plug, which prevents the female from remating before oviposition (Gilchrist and Partridge, 2000). Long-duration copulation could also reduce competition with previous ejaculates if males insert more sperm or spend longer removing their rival's sperm. According to Eberhard (1996) copulation duration may be a component of post-mating courtship, i.e., females may require some initial period of mating to evaluate the male before they permit the transfer of ejaculate.
It is known that female remating frequency in Drosophila depends on the amount of sperm stored (Manning, 1962; Gromko et al., 1984; Newport and Gromko, 1984; Letsinger and Gromko, 1985; Fukui and Gromko, 1989; Gromko and Markow, 1993), the components of the male seminal fluid (Wolfner, 1997), the level of nutrition and egg laying (Turner and Anderson, 1983; Harshman et al., 1988; Chapman and Partridge, 1996), testes size (Pitnick and Markow, 1994), body size (Pitnick et al., 1991), and on different experimental designs in the laboratory (Newport and Gromko, 1984). Several investigators have suggested that the frequency of remating is influenced by density. Boorman and Parker (1976) suggested that at higher population densities there is an increase in the incidence of courtship, resulting in a higher frequency of multiple mating in D. melanogaster, and Marks et al. (1988) presented evidence for a positive correlation between population density and remating in the field in this same species. However, increasing density decreased the frequency of remating in D. melanogaster (Gromko and Gerhart, 1984). Harshman et al. (1988) reported that the frequency of female remating in D. melanogaster was unaffected by density for some combinations of fly strains but was reduced at low relative densities for other combinations. In D. pseudoobscura and D. persimilis increasing density increases the frequency of female remating (Richmond, 1976; Levine et al., 1980). Aspi and Lankinen (1992) examined a natural population of D. montana and reported that low population densities at the end of the mating season probably force females to remate shortly after first mating. Ochando et al. (1996) found a high remating frequency (>50%) in D. melanogaster populations and suggested that population density may affect the incidence of remating in females. Recently, Singh and Singh (2001a) found that increased density increases the frequency of female remating in D. ananassae; they used two different experimental designs (2-h daily observation and continuous confinement). Correlations between a low incidence of remating and brief duration of male-female interaction (Manning, 1962) and between a high frequency of remating and uninterrupted confinement of the sexes together (Lefevre and Jonsson, 1962; Prout and Bundgaard, 1977) has repeatedly been found in D. melanogaster. Since the quantity of sperm used by a female before remating is clearly affected by the amount of time elapsing between matings, the experimental design factors for obtaining and detecting rematings could have a substantial effect on the use of sperm from successive matings.
In D. melanogaster, there is a long delay in female remating after the transfer of a large amount of sperm (Letsinger and Gromko, 1985). However, Service and Vossbrink (1996) found that a longer delay in remating was associated with reduced usage of stored sperm. Remating is also delayed in lozenge mutant females of D. melanogaster, possibly because this mutation results in spontaneous ovulation, which may be confused with normal signals that cause the female to be refractory to mating (Fuyama, 1995). The ejaculatory-duct protein, esterase-6, secreted from the male accessory glands of Drosophila and transferred to females together with sperm during copulation is known to be responsible for sperm use, oviposition stimulation and suppression of female remating (Fuyama, 1995; Wolfner, 1997; Tram and Wolfner, 1998). Gromko et al. (1984) reported that females, as well as males whose sperm are in storage, benefit from some degree of delay in remating. However, the optimal delay is longer for males whose sperm are in storage than that for the females, because female reproductive success is decreased if remating is delayed until the supply of stored sperm falls below a minimum level (Pyle and Gromko, 1978). If the minimum, or threshold level is primarily female determined, a male's primarily avenue to increased reproductive success will be through an increase in the number of sperm transferred above the threshold number. These sperm are protected against loss due to female remating by the remating threshold. Several aspects of Drosophila biology support the idea that short, non-fertilizing sperm could function in sperm competition by serving as "cheap filler", to delay female remating (Snook, 1998). Short sperm are relatively cheaper to produce than long sperm since Drosophila males that produce larger sperm mature later than males that produce shorter sperm (Pitnick et al., 1995). Snook (1998) further suggested that oviposition is more important than sperm in delaying female receptivity to remating and that sperm hetero-genotypes may play a role in affecting female remating. van-Vianen and Bijlsma (1993) showed that female remating frequency is affected by the genotype of its first male mate, and suggested that this could be due to differences in the amount or quality of seminal fluid transferred during mating. Sgro et al. (1998) reported that the first male affects the time of remating in females. Newport and Gromkow (1984) and Gromko et al. (1984) have shown that females with less sperm in the first mating are more likely to remate. Similarly, Harshman and Prout (1994) proposed that variation among females for remating rates is due to the amount of sperm they are carrying from the first mating. Females that lay more eggs use up their sperm supplies more rapidly (Trevitt et al., 1988) and remate more often (Chapman et al., 1994). Chapman et al. (1994) found that when females were kept on "high-quality food", increased remating was detrimental in that it decreased life span and lifetime reproductive success of the females. The rate of remating was high on high-quality food as compared to low quality food (Gromko and Gerhart, 1984; Trevitt et al., 1988; Harshman et al., 1988).
Gromko and Markow (1993) demonstrated that females in the field remate in response to low sperm load. They also found that females that were receptive to remating produced fewer progeny than control females, i.e., the lower fecundity of the receptive females could be due to both partial exhaustion of the sperm storage and to displacement or inactivation of the seminal fluid that precedes sperm transfer (Harshman and Prout, 1994). Recently, Markow (2000) reported forced mating in natural populations of some species of Drosophila; this also influences female remating. Snook and So (2000) found that remating Drosophila females oviposited more eggs than non-remating females, and presented evidence that oogenesis and oviposition also triggers female remating, either alone or in concert with male effects. Recently, Mery and Joly (2002) studied multiple mating, sperm transfer and oviposition pattern in the giant sperm species, D. bifurca. They examined how sperm transfer varies as males mate with a series of females and how female receptivity changes with time after mating. And they found an extremely limited overlap of ejaculates due to i) reduced sperm transfer to females that had already mated, and ii) female remating depended both on the amount of sperm transferred and the modes of egg laying.
A number of hypotheses have been advanced to explain the origin and maintenance of remating behavior of female Drosophila. Remating may renew or replenish dwindling sperm supplies (Newport and Gromko, 1984). Remating by females is an insurance against male sterility and sub-fertility (Gibson and Jewell, 1982). Remating could increase the genetic heterogeneity of female offspring (Pyle and Gromko, 1978). Richmond and Ehrman (1974) postulated that it is advantageous for a female to remate and produce eggs of multiple male parentages. Anderson (1974) suggested that females need to replenish their sperm supply, after depletion due to egg laying. Boorman and Parker (1976) suggested that remating would also be favored if the first insemination is inadequate or if the energy cost to the female is greater for rejecting a persistent male than for remating. Competition between successive mates for the fertilization of females' eggs could result in male mating strategies, which could influence female remating (Manning, 1962; Parker, 1970). It has been observed in many species of Drosophila that one mating is not enough for lifetime fecundity and that remating is universally necessary for full fecundity and fertility in female Drosophila (Turner and Anderson, 1983; Markow, 1985; Ridley, 1988).
Post-mating sexual selection includes both male-male competition (sperm competition) and female choice (cryptic female choice) (Eberhard, 1996). Sperm competition is a widespread phenomenon, occurring in almost every species from C. elegans to human beings (Parker, 1970; Smith, 1984; Baker and Bellis, 1993; LaMunyon and Ward, 1999). Sperm competition is a type of "cryptic competition" because other types of competition, such as differences in mate searching, courtship ability and mate guarding, are easily observed (Gilchrist and Partridge, 2000). Sperm competition results in selection for both offensive and defensive male traits. On one hand, evolution will favor a male that can affect the storage of another male's sperm or that can use his own sperm in such a way that his own fertilization success is maximized (male adaptations for sperm priority). On the other hand, males that are able to prevent or reduce subsequent competition from sperm of other males (male adaptations to prevent females from remating) gain a selective advantage. The outcome of female remating will depend partly on how well these adaptations perform against each other (Parker, 1970). Parker (1970) argues that these two lines of adaptations will be in some kind of evolutionary balance. The total impact of sperm competition on male fitness (Gromko and Pyle, 1978) and the effect of remating on female fitness (Pyle and Gromko, 1978), together are an excellent example of sexual selection. Sperm competition offers a unique opportunity to study adaptations shaped by the interacting forces of natural, sexual and antagonistic selection (Rice, 1996). The existence of sperm competition in Drosophila has been inferred from the proportion of progeny produced by the second male in double mating experiments (Nonidez, 1920; Lefevre and Jonsson, 1962; Clark et al., 1995; Singh and Singh, 2001b). This approach has been used to quantify genetic variation based on sperm competition (Hughes, 1997; Clark and Begun, 1998), to elucidate its genetic basis (Civetta and Clark, 2000a), to identify the dependence of differential male competitive ability on the genotype of the females with which they mate (Clark et al., 1999), and to discern the potential role of sperm competition in species isolation (Price, 1997; Price et al., 2000).
Sperm competition arises mainly because of the elaborate capacity for sperm storage within the female, because of the highly efficient use of stored sperm at fertilization, and because of the high probability of remating (Parker, 1970). Sperm competition may be intense when the sperm of several males is stored simultaneously within specialized storage organs or parts of the female reproductive tract before fertilization (Simmons and Siva-Jothy, 1998). Traditionally, sperm competition has been seen as an intra-sexual conflict, with females being an inert arena in which the conflict occurs. However, experimental evidence has shown that the manipulating role of females may be substantial and can have a dramatic effect on the outcome of sperm competition games (Parker, 1998). In the extreme case, it has been suggested that females may mate with a number of males and then select the sperm that will be used to fertilize their eggs (cryptic female choice (Eberhard, 1996). The female selects sperm through spermicide, be this by discarding received sperm, by digesting sperm or through phagocytosis of spermatozoa (Greeff and Parker, 2000). It has also been conjectured that males have evolved to produce large quantities of sperm in order to confuse or otherwise confound female's cryptic system of selection. Spermicide may benefit the female simply by providing a valuable nutrient source (Walker, 1980).
Sperm competition theory predicts that males adapted for success in sperm competition by producing large numbers of sperm. This is supported by both inter- and intra-specific studies, showing that males mating under high sperm competition risk increase investment in sperm production. This means that males whose sperm is at risk of competition should release more sperm during copulation than males whose sperm are not at risk. If the number of sperm released is unimportant to the outcome of the competition, then males should release the same number of sperm regardless of the risk of competition. An increase in sperm production would be an advantage if sperm mix randomly or if sperm displacement occurs (Cook et al., 1997).
What determines which sperm are used at fertilization has been extensively studied, and many male adaptations that promote fertilization success have been identified (Gromko and Pyle, 1978; Smith, 1984; Birkead and Møller, 1998). However, the copulating male alone does not determine the outcome of sperm competition. If we assume the most common form of sperm competition, in which two males inseminate a female, then the outcome depends on the morphological, behavioral, physiological and anatomical characteristics of i) the first male to mate, ii) the second male to mate, and iii) the female, including the biological properties of the ova. Thus, variation in the morphology and physiology of females and the ova is likely to influence the success of a male's sperm under conditions of sperm competition (Eberhard, 1996).
Male adaptations that increase the probability that a female inseminates her eggs with his sperm, and not the sperm of some other male, will be favored by selection (Gromko and Pyle, 1978). Such adaptations mainly include behavioral, physiological and anatomical characteristics of the male as well as competitive properties of the sperm itself. Examples of such adaptations include: i) pre- and post-copulatory guarding behavior, ii) mating plugs, iii) chemical or physical characteristics of the ejaculate which reduce receptivity to remating, iv) sperm displacement (i.e., the replacement by a subsequent mating of the stored sperm of a previous mating or sperm pre-dominance or sperm precedence of second male to mate), which could be affected either by the male during copulation or by some competitive ability of the ejaculate, and v) sperm precedence (i.e., non-random use).
How can a male ensure that his sperm will be the most likely to fertilize the female's eggs? One way is simply to monopolize the female so that other males do not have access to her. Male adaptations for sperm priority include: sperm removal, in which males manipulate the fertilization success by mechanical sperm removal, flushing the earlier male's sperm, and chemical sperm removal (Parker, 1970; Harshman and Prout, 1994); stratification of sperm (Pitnick and Markow, 1994); sperm dilution (Parker et al., 1990); manipulation of the fertilization process (Eberhard, 1996) and sperm size and sperm dimorphism (Pitnick and Markow, 1994; Snook, 1998). Male adaptations to prevent females from remating include: mating plugs (Parker, 1970; Polak et al., 2001), prolonged copulation and post-copulatory guarding behavior (Gilchrist and Partridge, 2000), and male-induced female non-receptivity (Eberhard, 1996). Recently, Hunter and Birkhead (2002) tested the hypothesis that sperm viability, measured as the proportion of live sperm, co-varied with the intensity of sperm competition in insects. In pair-wise comparisons of seven closely related species pairs, including D. pseudoobscura, each comprised of a monoandrous and a polyandrous species (with and without sperm competition), Hunter and Birkhead (2002) found that in all cases the polyandrous species had a higher proportion of live sperm in the sperm stores, suggesting that males can vary in their ability to fertilize ova on the basis of sperm viability alone. Furthermore, they suggested that sperm viability is among a suite of male adaptations to sperm competition in insects.
Sperm competition is also reported in human beings, which has become a recent focus of evolutionary biologists and evolutionary psychologists (Baker and Bellis, 1988, 1993). Baker and Bellis (1993) documented that male humans, like male insects, male birds, and other male non-human primates, appear to be physiologically designed to solve the adaptive problems of sperm competition. Baker and Bellis (1993) studied the risk of sperm competition as a proportion of the time a couple has spent together since their last copulation. They further documented a large positive correlation between the risk of sperm competition and the number of sperm a male inseminates into his partner at the couple's next copulation. In 1988, Baker and Bellis first proposed the "Kamikaze sperm" hypothesis. This was an extension of Parker's ideas (1970) on the role of mating plugs and blocking agents in sperm competition. In essence, they envisaged two types of human sperm, "egg-getters" and "Kamikaze" forms, specialized either to block competitors or to roam around the female tract seeking to destroy competitors (using acrosomal enzymes).
In humans, the female orgasm has been suggested as a means by which humans undergo sperm competition. The "upsuck" hypothesis for the function of the female orgasm has to do with an increase in the number of sperm taken into the uterus (Baker and Bellis, 1993). Uterine contractions during orgasm are suggested to increase the likelihood of conception (Baker and Bellis, 1993). Female orgasms occurring within one minute after the male's ejaculation had the highest rate of sperm retention by the female (Baker and Bellis, 1993). Females should therefore be able to either subconsciously or consciously alter the timing and type of orgasm to favor the retention of a specific male's sperm (Baker and Bellis, 1993). Males also demonstrate some aspects of sperm competition: when perceived risk of extra pair copulations is high (when the two partners spend little time together) males inseminate more sperm into the female at each copulation than when the perceived risk of sperm competition is low (Baker and Bellis, 1993).
A recent study (Wyckoff et al., 2000) reports that genes governing human sperm production evolve more quickly than other human genes. These findings suggest that at some point human females engaged in sex with multiple partners often enough to force sperm cells from different males to compete against one another to inseminate the egg - a hypothesis that may improve our general understanding of evolution. Our understanding of evolution is based on two concepts: natural selection and sexual selection. Natural selection, as it is described by Charles Darwin's book On the Origin of Species, occurs because species that are the best adapted to environmental strain are genetically more likely to survive than maladapted species - a theory he called "survival of the fittest." Sexual selection, which Darwin discusses in his book The Descent of Man, and Selection in Relation to Sex, is the theory that genes whose carriers are more capable of attracting members of the opposite sex are more likely to be passed from one generation to the next. But Wyckoff et al. (2000) point out that these forces can be at odds with each other. For example, the male peacock has a colorful tail that attracts females. Although this is beneficial with respect to sexual selection, it is disadvantageous in terms of natural selection because it makes the peacock an easy target for its predators. The recently discovered rivalry between different sperm cells inside the female reproductive tract may play a role in the genetic competition between men, and consequently may be a key to a better developed understanding of sexual selection and its role in human evolution. In their view, "Sexual selection can be counter-balancing to the emphasis of survival of the fittest". They indicated that human sperm and chimpanzee sperm evolve faster than gorilla sperm. Chimpanzees are, "highly promiscuous," and their sperm cells are strongly competitive. On the other hand, gorillas, which procreate according to a harem structure in which females do not mate with multiple males, do not have such highly competitive spermatozoa. They have analyzed the data in order to determine whether these differences in evolution rates are due to the existence of advantageous mutations or a lack of disadvantageous mutations. According to Wyckoff et al. (2000), the fact that chimpanzee sperm evolve faster indicates that the sperm must be under some sort of evolutionary pressure. This pressure is most likely caused by natural or sexual selection. The similarity between human and chimpanzee sperm evolution may indicate that these two species have similar social-sexual structures.
MALE ACCESSORY GLAND SECRETION AND FEMALE REMATING
There are two accessory glands in the reproductive tract of Drosophila males, and there are two types of secretary cells in these glands. These cells are predominately "main" cells, which comprise about 90% of the secretary cells and the rest are "secondary" cells. Each cell type produces specific types of proteins, beginning at eclosion (Wolfner, 1997). The mature adult male maintains constant levels of accessory gland proteins (Acps). Following mating, the level of gene expression in the main cells of the accessory glands increases, to replenish the secretions the male has just transferred to his mate (Wolfner, 1997). Replenishment of the secretions is important because multiple matings in rapid succession deplete the accessory gland contents, resulting in impaired fertility (Lefevre and Jonsson, 1962). The male ejaculate contains not only sperm, but also many nutrients and chemical substances that may be subjected to selection through sperm competition. Accessory gland proteins and ejaculatory duct proteins in the seminal fluids transferred to the female during mating have important reproductive functions, including increased oogenesis, ovulation, oviposition rates (Wolfner, 1997), decreased receptivity to male courtship (Wolfner, 1997), mediation of sperm storage (Neubaum and Wolfner, 1999), sperm competition (Civetta, 1999), and decreased life span (Chapman et al., 1995; Lung et al., 2002). Drosophila males also transfer antibacterial protein from their accessory glands and ejaculatory ducts to their mates (Lung and Wolfner, 2001), which help protect the male's reproductive tract and, after mating, the female reproductive tract or eggs against bacterial infection. They also protect the sperm because the male transfers the seminal fluid proteins before the sperm. The ejaculatory duct protein esterase-6 is transferred to the female in the seminal fluid, and is reported to affect sperm use and progeny production (Wolfner, 1997). There are eight types of seminal fluid proteins reported for Drosophila (Lung et al., 2002). The accessory gland proteins fall into three structural classes: i) simple peptides, which mediate changes in egg laying and receptivity, ii) prohormone-like and other larger molecules such as Acp26Aa and Acp76A that stimulate egg laying on the first day after mating, and iii) a large glycoprotein, such as Acp36DE, that may function in sperm storage or utilization. The Acp36DE also plays a role in "corralling" the sperm, reducing sperm wastage and assuring that a greater number of sperm enter the storage organs of the female. The proteins Acp36DE, Acp26A, Acp29AB, and Acp53Eb are involved in the management of sperm that is transferred from male to female, sperm storage, sperm displacement and reproductive isolation (Wolfner, 1997; Neubaum and Wolfner, 1999). Recently, it was reported that Acp62F is toxic to females following copulation, leading to a decreased female life span (Lung et al., 2002). Acps also act as agents for the incapacitation of sperm stored in the female's sperm storage organs in Drosophila (Harshman and Prout, 1994; Clark et al., 1995) and they play a role in cryptic female choice (Eberhard, 1996) and inter-sexual genomic conflict (Rice, 1996). Thus, Acps seem to be a currency of chemical communication between males and females (Wolfner, 1997).
SPERM PRIORUTY: SPERM PRECEDENCE OR SPERM DISPLACEMENT
The existence and relevance of sperm competition in Drosophila has been a contentious issue in evolutionary genetics (Hughes, 1997). Some of the available evidence suggests that female Drosophila remate only when they have depleted their reserves of stored sperm (Manning, 1962; Gromko and Pyle, 1978; Gromko and Markow, 1993). Under these conditions, competition between gametes from different males will have little effect on male reproductive success. However, other studies suggest that females readily remate before sperm stores are depleted (Gromko et al., 1984; Harshman et al., 1988; Clark et al., 1995). In this case, relative fertilization success is an important aspect of male fitness and should be subject to strong directional selection. After remating it has been observed that sperm from the last (or second) male usually take precedence over those of previous males (Boorman and Parker, 1976; Harshman and Prout, 1994; Clark et al., 1995; Hughes, 1997; Singh and Singh, 2001b) and preferentially fertilize subsequent eggs. More than eight decades ago, this phenomenon, now known as sperm displacement or sperm predominance or sperm precedence of second male to mate, was discovered in Drosophila melanogaster by Nonidez (1920). Lefevre and Jonsson (1962) directly observed sperm displacement in the female reproductive organs of D. melanogaster and suggested that circulation of sperm within the uterus or movements of the female reproductive tract provoke displacement. A reduction in the reproductive success of the first male occurs even if the second male transfers only seminal fluid and no sperm (Harshman and Prout, 1994). Seminal fluid proteins therefore play a role in second male sperm precedence (Wolfner, 1997), but the extent of this role is unclear because some studies (Lefevre and Jonsson, 1962; Gromko et al., 1984) have found no seminal fluid effect. However, Price et al. (1999) followed GFP-labeled sperm in doubly mated females and found that males can both physically displace and incapacitate stored sperm from earlier-mating males. Displacement occurs only if the second male transfers sperm to the female, and in only one of her three sperm storage organs. Moreover, either fertile or sperm-less second males, that requires extended intervals between mating can cause incapacitation. Sperm from different males are not 'stratified' in the storage organ, but mix freely. Further, they also found that the seminal fluid of a second male inhibits the use of stored sperm without removing them. Seminal fluid proteins are highly variable and are involved in a male-female co-evolutionary arms race (Civetta and Singh, 1995).
The phenomenon of sperm competition, which leads to sperm displacement, has been studied by numerous investigators in both natural and laboratory populations of different Drosophila species (Lefevre and Jonsson, 1962; Boorman and Parker, 1976; Cobbs, 1977; Prout and Bundgaard, 1977; Loukas et al., 1981; Griffiths et al., 1982; Gromko et al., 1984; Turner and Anderson, 1984; Barbadilla et al., 1991; Joly et al., 1991; Aspi, 1992; Aspi and Lankinen, 1992; Harshman and Prout, 1994; Clark et al., 1995; Ochando et al., 1996; Prout and Clark, 1996; Price, 1997; Hughes, 1997; Clark and Begun, 1998; Price et al., 1999; Bundgaard and Barker, 2000; Gilchrist and Partridge, 2000; Civetta and Clark, 2000a; Singh and Singh, 2001b). It is also reported from various insect species (Reinhardt, 2000), in birds (Birkead and Møller, 1998) and in mammals (Huck et al., 1985). Sperm displacement has been studied in more that 60 species of insects and in approximately 75% of these the last male to mate gains a paternity advantage beyond that expected on the basis of the relative number of sperm contributed to the fertilization pool (Ridley, 1988; Simmons and Siva-Jothy, 1998). Sperm competition can have significant effects on male fitness. Sperm competition has centered around the question of whether females tend to remate only after most of the stored sperm have been utilized, i.e., "sperm dependence" of remating (Manning, 1962; Gromko et al., 1984; Newport and Gromko, 1984), or whether remating occurs relatively rapidly and before first male sperm have been substantially depleted (Lefevre and Jonsson, 1962; Prout and Bundgaard, 1977; Harshman et al., 1988). The sperm competitive ability includes sperm defense. The defensive ability of a first male might be quantified by the likelihood that a female will remate before using all of his stored sperm. Females mated to first males with greater defensive ability will not remate until fewer sperm remained in storage. The sperm defense capability of a first male might further be quantified by the ability of his sperm to resist displacement or to reduce the fertilization success of second male sperm. There is also sperm offense. The offensive capability of the second male could be quantified by his ability to induce a female to remate before she has utilized all stored sperm and given that the female remates, offensive capability might be quantified by the ability of the second male to displace the sperm of the first male. From a fertility fitness perspective the greatest male fitness would come from a genotype that maximizes the number of offspring fathered, regardless of the order of mating. If we assume that multiply-mated females have mated with only two males, the total sperm competition fitness component for males is dependent on three parameters; P', P1 and P2. P' is the number of offspring fertilized by a male genotype after a female remates; P1, when he was the first male and P2, when he was the second male (Boorman and Parker, 1976; Gromko et al., 1984; Turner and Anderson, 1984). From a fitness point of view, the important parameter is the total of all three, which gives an average number of offspring, fertilized by a particular male genotype. However, Clark and Begun (1998) suggested that P2 measures the fraction of offspring sired by the second male when the matings are observed. They have given a new statistic P2', in which matings are inferred to occur from the offspring counts. The formula by which the second male precedence (P2) can be calculated is as follows:

where AFT1 is the number of progeny sired by first male after remating and AFT2 is the number of progeny sired by the second male after remating (Letsinger and Gromko, 1985; Singh and Singh, 2001b). The P2 values for different species are given in Table 2. The number of progeny sired by the first male after remating, and the second male progeny before and after remating are given in Figure 1, which is based on the work of Singh and Singh (2001). According to Parker (1970) the P2 value is important in determining evolutionary adaptation to the selective pressure of sperm competition. The proportion of progeny sired by the second male to mate (P2) in different species is given in Table 2.
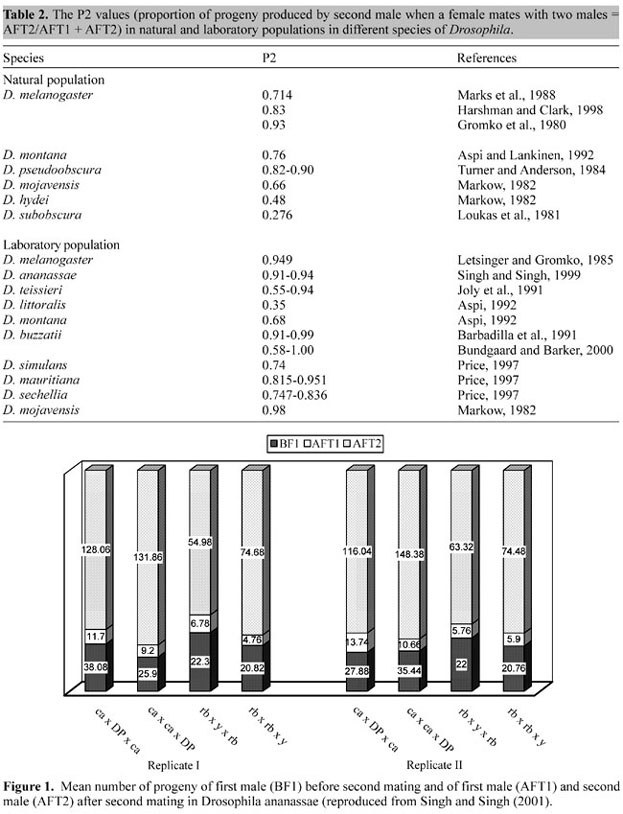
There are two terms frequently cited in the literature, which are involved in the sperm competition process; these are sperm precedence and sperm displacement or sperm predominance. Sperm precedence refers to the proportion of progeny from a remated female that are fathered by each male. However, a preponderance of offspring from the last male has been termed sperm displacement or sperm predominance. Sperm precedence is simply the non-random utilization of sperm from one of the two males to mate with a female. This is a passive process where sperm mixing is negligible, so that the sperm from different males become stratified within the spermatheca of the female. The last sperm to enter the female's sperm storage organ may be the first to leave, yielding a high value of P2. However, sperm precedence can also occur when the sperm from the first male are the first to leave the spermatheca, resulting in very low value of P2. In contrast to sperm precedence, sperm displacement is the active removal of previously stored sperm by the copulating male, either by flushing the female's sperm storage organs with his ejaculate or by mechanically removing sperm prior to ejaculation. P2 values are generally used to infer the mechanisms underlying the patterns of sperm utilization; intermediate values are taken as indicative of sperm mixing while high P2 values are taken as evidence for sperm precedence of the second male, or sperm displacement. We assume that when P2 values are high, sperm competition would be intense. However, P2 would be high whenever sperm are lost or removed from the female's sperm storage organs prior to or during the second mating. In this case, the sperm of successive males are not present within the reproductive tract of the female and do not compete for fertilization. Sperm competition occurs only when sperm from the two males mix within the female's sperm storage organs either immediately following mating or after sperm precedence mechanisms break down. Thus, sperm competition is likely to be at its most intense when intermediate values of P2 are observed (Simmons and Siva-Jothy, 1998). This is an important point because adaptations such as increased testes size or ejaculate size have been predicted to arise when sperm are in direct competition (Parker, 1993). Low P2 values have been observed when the second male to mate pre-empts the sperm from previous males through sperm displacement or precedence, or when the female's sperm storage organs are depleted prior to the second mating. Remating is provoked by inadequate insemination by the first male, which is further supported by the fact that low numbers of sperm available from the first ejaculate leads to a high predominance of the second ejaculate at fertilization (Boorman and Parker, 1976).
Two main techniques have been used to measure sperm utilization patterns in Drosophila: i) irradiated male techniques (Boorman and Parker, 1976), and ii) genetic markers such as color variants (mutants), enzyme polymorphisms, inversion lines, chromosome substitution lines, etc. Recently, some molecular techniques (molecular markers) have been employed to determine the sperm utilization pattern in Drosophila (Imhoff et al., 1998). Clark et al. (1995) measured genetic variation in the male component of sperm displacement by scoring progeny from a large number of females doubly mated with a tester male and males from a series of 152 chromosome replacement lines. In their view, P2', the fraction of progeny sired by the second male, varied widely, ranging from 1.0 (complete second male precedence) to 0.5. In addition to this "offensive" component, Clark et al. (1995) found genetic variation in the ability of first mated males to "defend" against displacement from subsequent matings. Hughes (1997) also found differences among males in both first and second male precedences among isogenic chromosome III lines extracted from a laboratory population; however, the variation was primarily non-additive. He further suggested that a few genes with a strong homozygous effect have an influence on sperm precedence. Clark and Begun (1998) found extensive genetic variation among females from different chromosome extracted lines in their ability to differentially use two alternative sperm sources, and they suggest that females also have a role in determining which sperm is used during fertilization. Females may influence paternity by extruding or digesting sperm of different males selectively by using adaptations in the physiology, anatomy and function of their sperm storage organs (Eberhard, 1996), sometimes in combination with behavioral adaptations. The impact of sperm storage as part of the female reproductive strategy depends on how their variability is maintained, and how they are released at fertilization (Pitnick et al., 1999; Neubaum and Wolfner, 1999). However, Clark et al. (1999) found that the chances of a male fertilizing the female's egg are dependent on both male and female genotypes, suggesting that female x male interactions play an important role in sperm precedence. Civetta and Clark (2000a) found significant male and female effects on sperm precedence. Furthermore, they also found that male and female characteristics independently influence fertilization success, suggesting that the variation found in P1 and P2 has a different genetic basis in the two sexes. Civetta and Clark (2000b) tested the relationship among the longevity of females mated to males from 51-chromosome extracted D. melanogaster lines, male mating ability, and sperm competitive ability, and found a trade-off between the defensive sperm competitive ability of males and life history parameters of mated females.
Ridley (1989) listed 57 insect species for which there is evidence of sperm displacement. He compared four, not mutually exclusive, hypotheses to explain why the degree of sperm displacement is greater in some than in other species. The hypotheses include: species with mating plugs have low sperm displacement and species without plugs high sperm displacement (Boorman and Parker, 1976); species with spheroid spermatheca have low sperm displacement and species with elongate spermatheca have high sperm displacement (Walker, 1980); species with low paternal investment have low sperm displacement and species with high paternal investment have high sperm displacement (Gwynne, 1984); and species with high mating frequencies have high sperm displacement (Ridley, 1989). Ridley (1989) concluded that evidence favors the "mating frequency hypothesis": species with a high mating frequency have a large degree of sperm displacement. Simmons and Parker (1992) have given a model of sperm displacement in which sperm mix quickly upon introduction to the sperm stores, and suggested that "large" males have a greater rate of sperm displacement than "small" males. However, males that copulate longer displace a greater number of rival sperm (Parker, 1970), because longer copulation results in the transfer of greater quantities of sperm. Harshman and Prout (1994) reported sperm displacement without sperm transfer in D. melanogaster in two different kinds of experiments. In one set of experiments, mated females were remated to two different kinds of sterile males, one with normal semen and another with deficient sperm. A second set of experiments employed interrupted mating and the results of these two experiments clearly indicate that a sperm incapacitation process plays a role in the well-documented phenomenon of sperm displacement (last male advantage) in this species. Further, they concluded that a seminal fluid agent is involved in sperm displacement, which strongly supports the view that this displacement process is a direct male "adaptation" resulting from sexual selection among competing males for those that best displace the resident sperm. However, Newport and Gromko (1984) reported that the replacement of first male sperm occurs as a by-product of the process of female storage and use, and not as a consequence of any particular adaptation of the second male to get rid of previously stored sperm. Barbadilla et al. (1991) studied female remating and sperm displacement in D. buzzatii and found strong sperm displacement (P2 = 91-99%). In the same species Bundgaard and Barker (2000) found strong sperm displacement, also with considerable variation between different combinations of first and second males. In some combinations they found complete sperm displacement (P2 = 1.00) and suggested that the mechanism responsible for sperm displacement can be very precise. Varying the time between the first and second mating did not change P2 and also sperm displacement did not depend on the number of previous matings (Bundgaard and Barker, 2000). Turner and Anderson (1984) measured sperm predominance after remating in D. pseudoobscura karyotypes carrying various combinations of four inverted gene arrangements in which sperm predominance depends on genotypes of males and females did not significantly affect sperm predominance. Further, they found P2 values varying from 0.82 to 0.90 for the second male and proposed that sperm predominance depends on whether the males are good or bad "resisters" of displacement or second male predominance, rather than good or bad "displacers".
The experimental design may also affect the outcome and can produce considerable variation in experimental results of sperm displacement. Newport and Gromko (1984) proposed that experimental design might affect sperm priority in Drosophila. They further proposed that female remating in a periodic design depends on the amount of sperm transferred and in continuous confinement female remating is rapid because the female is forced to remate with a second male, hence there is no sperm dependence. The time interval between matings may also influence sperm displacement (Boorman and Parker, 1976; Ridley, 1989). There are examples of both increasing and decreasing last male precedence with a longer mating interval. When P2 increases during a long time interval, one possible explanation would be that females run out of sperm over time, resulting in high fertilization success by the latest male when remating occurs (Ridley, 1989). If P2 decreases with an increase in the mating interval, it may due to sperm migration. When sperm have reached the spermatheca the last male may not be able to remove the sperm.
Sperm precedence, or nonrandom differential fertilization success among males, can be an important component of sexual selection in species in which females mate with more than one male (Parker, 1970). There are several potential mechanisms for sperm precedence. It could be due to a numerical advantage, if sperm competition is like a raffle (Parker, 1970). It could also occur if there is differential fertilization capacity resulting from variations in sperm motility, longevity, or ability to penetrate the egg. Effects of sperm number and differential fertilization capacity are analogous to male-male competition. Cryptic female choice, or biased sperm use from preferred males, could also affect sperm precedence (Eberhard, 1996; Birkead and Møller, 1998). Body size, and ejaculate size (Pitnick and Markow, 1994) also affect sperm displacement in Drosophila. Larger males have a greater share of paternity than their smaller rivals. This may occur because the larger males fill the spermathecae of the females more extensively than the smaller males, thereby leaving less volume for the smaller males (Simmons and Siva-Jothy, 1998), and or females may preferably store and use the sperm of larger males. Alternatively, larger males may be able to monopolize females when they are more receptive. Thus, selection may favor larger body size in species where sperm competition is intense. The functional significance of sperm morphs and their role in sperm competition has been studied (Joly et al., 1991; Snook, 1998). Both sperm morphs (short and long) are involved in the fertilization process (Joly et al., 1991); however, Snook (1998) showed that only long sperm participate in fertilization in D. pseudoobscura. This dimorphism in sperm is a male adaptation, i.e., long sperm would be favored when males mate first with females that will mate again later (Joly et al., 1991). The advantage enjoyed by second males suggests that sperm from some males are more likely to fertilize eggs, regardless of their numerical representation. This could be due to differential fertilizing capacity if "good maters" produce more competitive sperm. Males with different genetic backgrounds appear to have different abilities to induce sperm displacement (Clark et al., 1995). And yet this trait appears to have very low heritability (Gilchrist and Partridge, 1997). Differential fertilizing capacity and preferential movement of sperm by females is analogous to male-male competition and female choice, except that they act at the gametic rather than the organismal level.
MECHANISM OF SPERM PRECEDENCE OR DISPLACEMENT
The mechanism by which, sperm displacement is accomplished is still unknown. However, some investigators are trying to investigate this. The mechanism by which the sperm of one male is more successful in fertilization than that of another is often assumed to be species specific (Birkead and Møller, 1998). Five models of last-male sperm precedence mechanisms have been proposed and one way of testing these models is by analyzing the variation in P2 values with time (Birkead and Møller, 1998; Simmons and Siva-Jothy, 1998; Parker, 1998). In the first, the "sperm-mixing model ", a complete and instant mixing of two ejaculates is assumed. The P2 value remains constant at about 50%. In the second, the "sperm stratification model ", sperm of one male (the second) is situated closer to the fertilization site than that of the other (the first male). In this case the P2 value will be near 100% until the sperm from the second male is completely used up, when the P2 value suddenly drops towards zero while P1 rises to 100% (Birkead and Møller, 1998). In the third, the "sperm-repositioning model ", the sperm of the second male lies closer to the fertilization site but the two ejaculates start to mix immediately. In this situation, the P2 value steadily declines from high values towards 50%. The fourth, the "sperm removal model ", predicts that the P2 value remains constant at the proportion by which the sperm of the first male were displaced. And in the fifth model, the "passive sperm loss model " (Reinhardt, 2000), the P2 value also remains at a certain high level. However, the sperm of the first male is not displaced by the second male, rather sperm is passively lost in the spermathecae of females. Parker et al. (1990) proposed a sperm displacement model, in which sperm displacement is viewed as a process in which one unit of introduced sperm displaces one unit of sperm from the female sperm stores. They developed prospective models based on two principle competitive mechanisms: a "raffle" (random sperm mixing), where sperm are equivalent to tickets in a lottery, and sperm displacement, where the male flushes sperm from the sperm storage organs using his own ejaculate. In the fair raffle, each sperm from each male has an equal chance of entering the fertilization set, which supports random sperm mixing. However, the sperm displacement model assumes fixed sperm storage capacity and no random mixing. The random sperm-mixing model assumes that the sperm storage organs are elastic according to the number of sperm stored after each mating. Parker and Simmons (1991) later redefined the displacement model, which assumed that sperm were not displaced on a one-for-one basis but rather on a volumetric basis, such that insemination involved a change in the density of sperm in the sperm storage organs. Recently, Harvey and Parker (2000) have developed a new model of sperm precedence "sloppy sperm model". This model assumes that it is the packets of sperm, rather than the sperm themselves, that are randomly mixed before fertilization.
There are only few cases in the insects, where the mechanisms of sperm displacement are given in detail. The first case includes damselflies and other odonates, in which there is mechanical removal of the previous male's sperm by specialized structures on the penis; the second case is the mealworm beetle (Tenebrio molitor) in which a penile sheath has reversed spines that scour out the previous sperm; the third case is a cricket (Triljalia hibinonis) in which the storage organs are filled by second male's sperm after mating and the previously stored sperm appears on the shaft of the second male's penis, indicating a flushing out process, and the fourth case is a carrion fly (Dryomyza anilis), in which the male massages the abdomen of the female, who then extrudes the previous male's sperm (Harshman and Prout, 1994, and references therein).
Turner and Anderson (1984) proposed three mechanisms that lead to differences in sperm predominance (high P2 value) in Drosophila: i) quality of ejaculate, that is a male with a larger quantity of sperm and seminal fluid may "flush" the female's reproductive tract of more stored sperm than a male with a lesser quantity of sperm and seminal fluid, ii) overall male vigor; this may overlap the first mechanism, since a more vigorous individual may produce more sperm and seminal fluid, and iii) the composition of the ejaculate. Two hypotheses have been proposed to account for the predominance of second male sperm and the loss of first male productivity when D. melanogaster females remate. According to the "incomplete storage hypothesis" (Lefevre and Jonsson, 1962): i) release of the first male's sperm from storage after remating due to the presence of second male sperm in the uterus; a physiological effect of second male seminal fluid or the act of copulation itself, and ii) incomplete sperm storage after each mating. The first males suffer a loss of productivity because their sperm undergo two cycles of incomplete storage. In contrast to the incomplete storage hypothesis, the "inefficient use hypothesis" postulates that sperm from a previous mating are not released from storage when a female remates (Newport and Gromko, 1984). Instead, the addition of second male's sperm to those already in storage initiates a second round of inefficient use, in which more sperm are released than would be necessary to fertilize the available eggs, so first male productivity is reduced by this second round of inefficient use.
Harshman and Prout (1994) have shown that seminal fluid from second males reduces the number of progeny sired by the first male, even when the second males are sterile, which demonstrates that a sperm incapacitation process plays a role in the well-documented phenomenon of sperm displacement in Drosophila. Price et al. (1999) reported that the seminal fluid of the second male inhibits the use of stored sperm (first male sperm) without removing them, leading to high P2 values (sperm precedence of second male to mate). Civetta (1999) used fluorescence microscopy, flow cytometry, and differently labeled sperm to analyze the fate of sperm inside the female's sperm storage organs, to quantify sperm competition, and to assess how closely paternity success corresponds to the appearance and location of the sperm. The results show that the first male's sperm is retained for a shortened period if the female remates, and that the second males that sire more progeny either induce females to store and use more of their sperm or strongly displace resident sperm. Gilchrist and Partridge (2000) investigated the effects of experimental manipulation of copulation duration on sperm displacement in Drosophila melanogaster by using spermless and normal males as second males. Displacement in duced in the absence of sperm, that is by males that pass accessory gland fluid alone, was found to be a relatively inefficient process and produced much lower levels of displacement than for normal males, and therefore the presence of second male's sperm is necessary for high levels of displacement in Drosophila.
An understanding of sexual selection in various species requires knowledge of events that occur after mating, and continued study of intra-specific variation and time-dependent changes in P2 will be useful in elucidating the mechanisms responsible for sperm competition.
GENETIC BASIS OF FEMALE REMATING IN DROSOPHILA
Experiments on the genetic basis of female remating in Drosophila have been mainly restricted to D. melanogaster. Gromko and his co-workers, using various experimental designs, have carried out most of the experiments on this in D. melanogaster. Their results vary with respect to remating speed (the time interval between the first and second matings). When they artificially selected single lines for fast remating in both sexes, a relatively small number of genes, deviations from additive gene action, and involvement of the X-chromosome were found (Pyle and Gromko, 1981). In selecting both fast and slow lines, which was based on behavior of one sex, they suggested that genes affecting remating speed in females are largely sex limited in their action (Gromko and Newport, 1988a). Moreover, Gromko and Newport (1988b) were able to demonstrate correlated responses to selection in virgin mating behavior, early fecundity, and courtship elicited by mated females, as well as the relation between sperm use and remating interval. Hudak and Gromko (1989) found positively correlated responses for fertility and remating speed in lines selected for speed of sexual maturity. However, chromosome substitution analysis, biometrical and planned comparison analysis, and recombination analysis of their experiments for remating speed demonstrate the involvement of chromosome II, which contributed significantly to the differences in remating speed in selected lines (Fukui and Gromko, 1991a,b,c). Stamencovic-Radak et al. (1993) reported that selection for females that remate readily could result in relaxed selection for male vigor in D. melanogaster. Sgro et al. (1998) found a significant direct response to selection for time to remating in D. melanogaster females for both selection lines (fast and slow) and suggested that remating time is heritable and that rapid remating is pleiotropically associated with frequent remating. They also suggest that remating time in females is under polygenic control. However, Singh and Singh (2001c) recently studied the genetic basis of female remating speed, for the first time in a species other than D. melanogaster, using bi-directional (fast and slow) artificial selection in D. ananassae, a cosmopolitan and domestic species which occupies a unique status in the genus Drosophila, owing to certain peculiarities in its genetic behavior (Singh, 1996, 2000). Response to selection resulted in a rapid divergence in remating time in each line. Their findings suggest that female remating time in D. ananassae is under polygenic control. The remating frequency of females showed a correlated response in both fast and slow lines, which suggests that remating time is heritable and rapid remating is pleiotropically associated with frequent remating in D. ananassae (Singh and Singh, 2001c). They also tested correlated responses to selection for mating propensity and productivity of single-mated females and found that fast lines had a positive effect on productivity and initial mating speed and slow lines had a significantly negative effect on productivity and mating speed. This shows that selection for remating speed resulted in correlated responses for mating activity and fertility in D. ananassae (Singh and Singh, 2001c). The results of the selection experiment for remating speed in both fast and slow lines for D. ananassae are given in Figure 2, which is based on Singh and Singh (2001c).
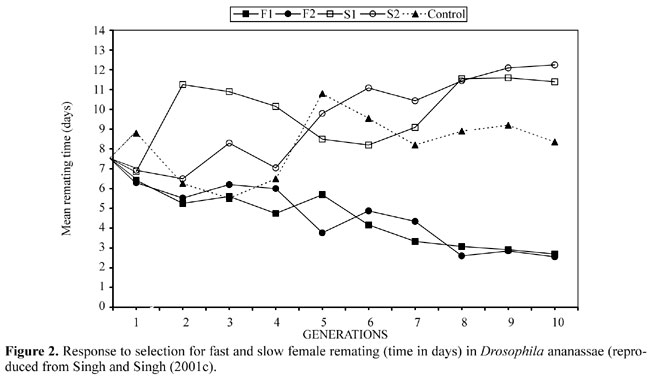
Several studies have suggested that genotypic variation for sperm precedence or sperm displacement exists both within and between populations. Prout and Bundgaard (1977) demonstrated that different laboratory strains of D. melanogaster have different degrees of sperm-competitive ability. There are some models of the population genetics of sperm displacement, which explain the maintenance of polymorphism of genes that influence sperm competition. Prout and Bundgaard (1977) presented a population genetics theory for sperm displacement, which is determined by a single locus with two alleles and found that a stable polymorphism in a sperm displacement locus can be maintained if the sperm displacement parameters are either over-dominance or non-transitivity of alleles. Prout and Clark (1996) have shown that pleiotropic effects on fecundity and male mating success can increase the opportunity for stable polymorphism for alleles affecting sperm precedence, but in this case induced over-dominance is neither necessary nor sufficient to guarantee polymorphism. Dominance variance was found to be significantly higher than additive variance for the offensive component of sperm displacement (P2), suggesting that genetic variation in this trait can be maintained by either over-dominance (Hughes, 1997) or by antagonistic pleiotropic interactions with other components of fitness. Recently, Clark et al. (2000) demonstrated that sperm competitive ability is not a property of sperm haplotype, but rather of the diploid male's genotype. They tested whether the relative sperm competitive ability of males can be ranked on a linear array or whether competitive ability instead depends on particular pair-wise contests among males, by using a set of six chromosome extracted lines. They found strong departures from transitive relations among a set of six genotypes of males as the pair-wise tests were not compatible with a single linear ranking of sperm competitive ability. Furthermore, they proposed that the non-transitivity seen in sperm precedence theoretically should increase the opportunity for polymorphism in genes that influence this phenotype.
FEMALE BENEFITS OF REMATING
Several satisfactory explanations for remating by females involve direct benefits. In many insect species, costs of multiple mating are offset via direct benefits due to: i) the replenishment of sperm stores or ii) nutrient donations found in the ejaculate from each of the mates. As such, the evolutionary maintenance of polyandry in insects can be understood merely in terms of direct benefits (although genetic benefits are not necessarily precluded). In some species, there may be no obvious benefit to females mating more than once, as since males force copulations it pays females to yield rather than waste time rejecting unwanted copulations. Yet, it has become apparent that in other species where there are no apparent direct benefits, females are actively soliciting and seeking copulations with multiple males, suggesting indirect benefits to multiple mating. In species where males contribute nothing more than sperm, the nature and existence of indirect benefits to females remains to be established and is currently one of the most important unresolved questions in sexual selection theory. Potential advantages of female remating for the females themselves can be assigned to one of two major categories: 1) direct (non-genetic) benefits including fertility benefits (i.e., enough viable sperm to fertilize all the eggs) and 2) indirect (genetic) benefits including benefits to offspring quality/quantity via greater genetic diversity in offspring or increased opportunity for female choice among sperm from different males of varying genetic quality (i.e., "good-genes"), or compatibility ("compatible genes").
There are few studies that clearly show evidence of female benefits through remating. Proposals for the adaptive value of female remating include: direct benefits, genetic benefits and life history benefits. Males of many species of Drosophila and other insects provide nutrition to females in some way (nuptial gift, male secretion during courtship or nutrition through ejaculates), which may represent a form of paternal investment to increase female reproductive success (Gwynne, 1984; Markow and Ankey, 1984; Markow et al., 1990; Pitnick et al., 1991). Snook and Markow (1996) found that these materials enhance female productivity over the first 10 days of life, but lifetime reproductive success was unaffected by levels of incorporation of males ejaculate. Snook (1998) suggested that non-fertilizing sperm serve as nutrient contributors and also function in sperm competition.
Several factors will determine whether males will be selected to employ this kind of reproductive effort: first, a male must be able to affect significant reproductive gain by virtue of his investment. Therefore, the nutritive component is expected to be a substance that limits the rate of reproduction by females. Consequently, the kinds of nutritive secretion contained within the ejaculate are expected to vary among species according to their unique ecology. Second, reinforcement of male nutritive investment requires adequate certainty of paternity. Markow and Ankey (1984) have demonstrated the potential for multiple mates of a female to cuckold each other's nutritive contribution in D. mojavensis. It is also true, however, that throughout the genus Drosophila the presence of ejaculatory donations is strongly associated with the formation of a copulatory plug (Polak et al., 2001), which presumably enhances a male's paternity assurance. Third, the reproductive costs, in terms of lost mating opportunities must be outweighed by the reproductive gains. This variable may differ among populations, primarily due to varying resource distribution and abundance (Chapman et al., 1995). The nutritive role of the male ejaculate has primarily been determined in experiments in which egg or progeny production is compared among single versus multiply mated females (Barbadilla et al., 1991; Clark et al., 1995; Bundgaard and Barker, 2000; Singh and Singh, 2001b), among females mated to virgin versus recently mated males that ejaculate but with no or depleted sperm (Markow et al., 1990); or among females that have been permitted to feed for different lengths of time on the spermatophylax or non-sperm containing portion of the spermatophore (Gwynne, 1984). Support for a nutritive role of male ejaculatory substances is strengthened by demonstrations that male-derived substances or their derivatives are incorporated into developing oocytes (Markow and Ankey, 1984; Pitnick et al., 1991). Polak et al. (2001) proposed that the mating plug in Drosophila, a firm, gelatinous structure that forms within the female's uterus during copulation, might serve as a nutritional gift that females digest, using the constituents for somatic maintenance or to provision eggs as they mature within the ovaries. Females may experience nutritional losses if males efficiently prevent remating. Also copulation may function as a payment for gaining access to some male-controlled resource. Females may also receive defensive substances from males during insemination, e.g., antibacterial protein from the accessory gland of male in Drosophila, which protects the reproductive tract of females and their eggs against bacterial infection (Lung and Wolfner, 2001).
Remating is an insurance against sterility and sub-fertility. Remating is necessary when a single insemination does not provide the female with sufficient sperm to insure high fertility during the female's reproductive period (Walker, 1980; Gibson and Jewell, 1982; Halliday and Arnold, 1987). The reason may be limited sperm storage capacity. This may be an economic optimization by which the female reduces the energy cost of keeping sperm alive for a long time (Ridley, 1988).
Genetic diversity in the progeny may be advantageous in unpredictable environments with strong sib-competition and multiple-mating females may then be favored over single-mating females (Maynard-Smith, 1956; Parker, 1970). However, by increasing genetic diversity in offspring, any restrains on sib-competition due to kin selection will weaken as well, and thus increase sib competition (Reynolds, 1996). According to Danielsson (1998) one mating would supply the female with a potential progeny diversity equivalent to one half of that of the whole population. A female could then, by mating with only a few males, obtain almost the total range of genetic diversity present in the population and should gain very little by further matings. Several hypotheses propose that the payoff to polyandry is genetically based. Genetic benefit hypotheses include: i) the trading-up hypothesis, that females in socially monogamous species engage in extra-pair copulations to compensate for a poor-quality mate (Ridley, 1993); ii) the offspring diversity hypothesis, that the increased offspring diversity resulting from multiple paternity enhances female fitness, either by reducing sibling competition or by serving as a hedge against environmental uncertainty (Keller and Reeve, 1995); iii) the intrinsic male quality hypothesis, that polyandry enables sperm competition or female choice of sperm to increase the probability that eggs are fertilized by high-quality sperm or sperm from high-quality males (Birkead and Møller, 1998); iv) the sexually selected sperm hypothesis, that genetic benefits derive from Fisherian sexual selection, by which the sons of multiply mated females produce competitively superior sperm or ejaculates (Keller and Reeve, 1995); v) the inbreeding avoidance hypothesis, that polyandry diminishes the cost of inbreeding in situations in which females cannot avoid mating with close relatives (Stockley, 1999); and vi) the genetic incompatibility avoidance hypothesis, that mating with several males enables females to exploit post-copulatory mechanisms to minimize the risk and or cost of fertilization by genetically incompatible sperm (Zeh and Zeh, 2001). Zeh and Zeh (2001) proposed that reproductive mode is a critically important factor influencing the type of genetic benefits that females gain by mating with more than one male.
If males differ in genetic quality, females will try to combine their own genes with male genes of as high a quality as possible. These high quality genes are called "good genes". The "good genes model" (Zahavi, 1977) states that females choose their mate, using male secondary sex traits as indicators that possess high genetic quality that will be passed on to their offspring. In this way the females that choose the most attractive (extreme) trait will produce more offspring or offspring of higher quality. When a female encounters a male that is better than the first male, she will remate and use the last male's sperm for fertilization (Eberhard, 1996). According to Hoenigsberg (1999), the good genes that are chosen by females may have an effect on either the viability or the mating success of their offspring. Further selection for the preference of this trait may be self-reinforcing and lead to runaway sexual selection in which the male trait becomes extremely exaggerated. Hoenigsberg (1999) reported that female competition in mate choice decreases the fitness of D. pseudoobscura flies. His results demonstrate that when mate choice is possible, there is a competitive success of offspring when compared to the offspring of females that do not choose their mates. He further proposed that when females receive genes from chosen mates their own additive fitness heritability makes choice worthwhile.
In species with sperm mixing (fair raffle game) females may devalue the sperm obtained by earlier mates by performing repeated matings with a preferred late male (Birkead and Møller, 1998). Yasui (1997) presented a "good sperm model" providing a mechanism for maintaining costly multiple mating by females. Multiple mating will induce sperm competition among a female's mates and the level of investment in the sperm competition trait determines male fertilization success. It was assumed that males with a greater general viability could invest more resources in sperm competition. Thereby multiple mating females receive good genes, because males with greater sperm competitive ability also possess far greater general viability. According to Danielsson (1998), if one compares two strategies producing the same mean number of offspring the strategy with the lower variance in offspring number will be favored. Female remating therefore has lower within generation variance in the expected number of offspring than in single-mating females. Female remating may, however, be favored in small populations or in populations that experience founder effects (Parker, 1970).
By remating and mixing the sperm of several males, females ensure that they are fertilized by the most competitive sperm, thereby increasing the probability that their sons also will have competitive sperm; this selective advantage after multiple mating is called the "sexy-sperm hypothesis" (Hoenigsberg, 1999). A similar argument can be used for paternity assurance mechanisms. Male paternity assurance mechanisms will prevent females from remating, but females will also benefit by having sons with effective paternity assurance mechanisms. Curtsinger (1991) studied a two-locus genetic model of the sexy sperm hypothesis and found that the conditions necessary for sperm competition to only promote multiple matings were rather restricted. According to Bernasconi and Keller (2001) the potential benefit of remating for females is the increased probability that their sons will inherit traits enhancing their pre- or post-mating ability to attain fertilization.
All biological processes directly related to reproduction play a role in determining fitness. Productivity is a major component of over all fitness in these processes, and in many circumstances it is the single most important one (Turner and Anderson, 1983). Female remating results in higher fitness in many species of Drosophila. Pyle and Gromko (1978), Clark et al. (1995) and McRobert et al. (1997) in D. melanogaster; Turner and Anderson (1983) in D. pseudoobscura; Barbadilla et al. (1991) and Bundgaard and Barker (2000) in D. buzzatii and Markow (1982) in D. mojavensis have reported a productivity advantage of multiply mated females over singly mated ones. Recently, Singh and Singh (2001b) also found greater productivity in remated females when compared to singly mated females in D. ananassae. However, Lefevre and Jonsson (1962) and Prout and Bundgaard (1977) found no difference between the productivity of singly and multiply mated females in D. melanogaster. Aspi (1992) did not find any difference between the productivity of once and twice mated females in the D. virilis species group. McRobert et al. (1997) reported no difference in the offspring production between D. biarmipes females allowed to remate and those once mated. Parker (1970) suggested that repeated mating by males is a form of sexual selection involving competition among males to mate, and it may bear little connection to adaptedness, or fitness of the population as a whole. However, repeated mating by females leads to higher productivity than mating just once, and it could easily be adaptive to the population in which it occurs by increasing the rate of population growth and population size, both measures of adaptedness (Parker, 1970). Recently, experimental studies by Arnquist and Nilson (2000), addressing the differential effect of multiple mating on female fitness, revealed that females generally benefit from remating in terms of increased lifetime offspring production.
The advantage of female remating is to guard against genetic incompatibility (Tregenza and Wedell, 2000). Genomes of species are dynamic entities constantly evolving as a result of genetic conflicts within and between the nucleus and the cytoplasm, called intra-genomic conflict (Rice and Holland, 1997). Selfish genetic elements such as cellular endosymbionts, transposable elements, segregation distorters, maternal effects, lethal and genomically imprinted genes, can modify maternal and paternal haplotypes enough to make them unable to combine and produce a viable zygote (Zeh and Zeh, 2001). Zeh and Zeh (2001) reported that remated females could minimize the risk or cost of being fertilized by genetically incompatible sperm, by post-copulatory mechanisms, e.g., sperm competition, sperm choice or reallocation of resources from defective to viable offspring. Females of many species may also remate to guard against abnormal chromosome numbers, defects due to long term sperm storage, or sterile or sub-fertile ejaculates (Halliday and Arnold, 1987). In addition, other mechanisms such as sexually antagonistic gene effects (Rice, 1996), the instability of hypervariable DNA (Zeh and Zeh, 2001), inbreeding depression (Stockley, 1999), and even the spontaneous tendency of sperm to take up foreign DNA and incorporate it in embryonic genomes (Zeh and Zeh, 2001), could all add to the disruption of genetic compatibility within the populations. Recently, a new benefit of female remating, reduction of maternal care, which leads to increases in the survival of females, has been reported in higher animals (Li and Brown, 2002).
MEIOTIC DRIVE AND FEMALE REMATING
Sperm competition has been defined as competition within a single female between the sperm from two or more males for the fertilization of her ova (Parker, 1970). In effect, sperm competition is seen as a continuation of inter-male conflicts within the female reproductive tract, which leads to sperm precedence or sperm displacement in many species of Drosophila. This definition ignores the possibility of competition among the haploid sperm produced by a single diploid male. If a sperm's phenotype were determined by the diploid genotype of its male progenitor, without influence from the particular genes that the sperm itself received, there would be no competition within an ejaculate and natural selection would act on the ability of entire ejaculates to increase the reproductive success of the diploid male. Differences in competition ability among the haploid genotypes present in the ejaculate of a single male are known as "meiotic drive" or "segregation distortion". Unambiguous examples of meiotic drive prove that a sperm's fate is not always independent of its own constitution. The meiotic-drive hypothesis posits the existence of a selectively maintained polymorphism, in which the segregative advantage of the driving allele is balanced by a viability advantage of the non-driving allele. Under this hypothesis, alleles that favor multiple mating become preferentially associated with the more competitive (non-driving) allele. Alleles for female remating can become fixed in the population because of heritable differences in the competitive ability of the sperm. Thus, the meiotic-drive hypothesis predicts that multiply mated females will have offspring with a higher average viability (Medsen et al., 1992). Haig and Bergstrom (1995) concluded that a gene that gains an advantage in a competition among the haploid products of a single diploid male may increase in frequency even if it has substantial negative effects on the fitness of the most of the other genes with which it is associate, and therefore predicted the promotion of mechanisms that limit the opportunities for successful meiotic drive. That is, most of the genome is unlinked to any particular driving locus and modifiers at unlinked loci are selected to reduce the intensity of meiotic drive. From this perspective, the diploid control of sperm function can be interpreted as an institution that suppresses competition within ejaculates for the greater benefit of the ejaculate as a whole. Similarly, if meiotic drive reduces the overall success of an ejaculate in competition with the ejaculates of the other males, then genes expressed in females that influence the probability of multiple mating are, in effect, modifiers of meiotic drive and will be selected to reduce the advantage enjoyed by the driving allele (Medsen et al., 1992).
SEXUAL CONFLICT AND FEMALE REMATING
In sexual reproduction there are two individuals who may actually have no genetic interest in each other's future, the parents, but who nevertheless have a joint genetic interest in the other individual or group of individuals. Remember that if investment to improve the prospect of the current offspring reduces the parent's own prospects (Lessels and Parker, 1999), then there will be a conflict between the two parents. A conflict that expresses courtship acceptance or refusal usually arises because each parent's fitness is generally maximized if it invests less and the other parent invests more than would maximize the other parent's fitness. Obviously, this conflict has been referred to as "the battle of the sexes". Selection for each parent to exploit the efforts of the other parent relies only on sexual reproduction and not on the existence of separate sexes. No doubt the conflict between parents that we study in behavioral genetics may be responsible for the evolution of sexes. Most of the conflict between mates is the result of post-copulatory sexual selection, that is sperm competition and cryptic female choice (Eberhard, 1996). Post-copulatory sexual selection generate sexual conflict through three discrete processes: i) there may be conflict over how many gametes are dedicated to each mate, called the "monopolization conflict" (Parker, 1970), in which there are paternity costs to males of female remating and fertility costs to females of males partitioning their sperm between successive mates. This type of conflict will generate selection for traits such as copulatory plugs (Polak et al., 2001), anti-aphrodisiacs and mate guarding by males, which invest in gametes monopolized through direct intervention of mate behavior; ii) conflict may evolve through physiological trade-offs between traits contributing to reproductive success called "allocation conflict"; this is common because one sex (females) invests predominately in offspring while the other sex (males) invests predominately in fertilization opportunities (Bateman, 1948), and iii) sexual conflict may arise when traits that are adaptive for one sex in reproductive competition have negative effects on the opposite sex called "by-product conflict" for example, the toxicity of male seminal fluid proteins in Drosophila (Chapman et al., 1995). Holland and Rice (1999) suggested that experimental manipulation of sexual selection provides a valuable approach for detailed investigations of sex-specific traits arising through allocation and by-product conflicts.
Partridge and Hurst (1998) reviewed how evolutionary conflict occurs when the deterministic spread of all alleles lowers the fitness either of its bearer or of other individuals in the population, leading to selection for suppressors. Sex promotes conflict because associations between alleles are temporary. Differential selection on males and females, sexual selection, and differences in transmission patterns between classes of nuclear and cytoplasmic genes can all give rise to conflict. Inert Y-chromosomes, uniparental inheritance of cytoplasmic genes, mating strains and sexes, and many features of sexual behavior may have evolved in part as result of evolutionary conflict. Any deviation from monogamy increases sexual conflict because an individual's lifetime reproductive interests will not coincide (Rice and Holland, 1997). Therefore, sexual conflict should increase with multiple mating (Rice and Holland, 1997), as does the potential for sperm competition. As a result, sperm competition should enhance sexual conflict and thus lead to the evolution of characters that increase reproductive success in one sex, while they are costly to the other. Also, when sperm competition is fundamentally analogous to a raffle, the relative number of sperm in competition will be the primary determinant of success (Parker et al., 1990).
There is an evolutionary arms race not only between sperm displacement and paternity assurance strategies in males, but also between male traits to achieve matings or to prevent female remating, and female counter adaptations or resistance to being manipulated (Rice and Holland, 1997). Two lines of argument may be used to predict the outcome of the sexual conflict: i) that those experiencing the strongest selection pressure will win the conflict, and ii) those that are in a superior position to manipulate the other will win the evolutionary race. The male will also feel any costs to females from mating with males through reduced reproductive success, which improves the competitive ability of sperm. Thus, selection for male paternity assurance is expected to be stronger than selection for female resistance to mating or male adaptations (Parker, 1970). On the other hand, since fertilization takes place within the female, she has the opportunity to control the reproductive processes and influence sperm use (Eberhard, 1996). Several female counter-adaptations for reducing male-imposed limitations to their reproductive success have been proposed. For example, females may lower their conspicuousness to males by physically resisting mating attempts, they may possess specialized organs for rejecting males, remove mating plugs, disguise their reproductive status and place barriers in the reproductive tract to resist overly aggressive sperm (Eberhard, 1996; Stockley, 1999). When selection acts in separate directions on a homologous character in males and females, a genetic correlation between the sexes for this trait will slow down the rate of divergence and an evolutionary optimum may never be reached. For instance, if there is a genetic correlation between the sexes in mating frequency, females may mate repeatedly as a correlated response to stronger selection on the male to do so. Rice (1996) presented empirical evidence for an evolutionary arms race between the sexes using the genetic features of D. melanogaster. He used an artificial experiment in which females were prevented from co-evolving with males. Males then rapidly adapted to the static female phenotype and increased their ability to remate with non-virgins, to prevent the female from remating, and to displace rival sperm. This increase in fitness of the males was accompanied by a decrease in survival of their mates, suggesting counteracting adaptations in the two sexes. Recently, Pitnick et al. (2001) revealed that sexual selection favors larger males, which invest a greater production of their total energy product in sperm production. While observing the greater reproductive success of females with monogamous-line males they suggested that male and female reproductive interests do not naturally counteract in D. melanogaster.
IS REMATING COSTLY TO FEMALES?
It has been observed in Drosophila and in many other insects that sexual activity and mating are costly to females in terms of future survival (Ikeda, 1974; Turner and Anderson, 1984; Chapman et al., 1995; Sgro and Partridge, 1999; Lung et al., 2002). But many females also mate repeatedly with different types of males, including poor quality males. So if remating is costly why do females continue to mate with poor quality males? There are two possibilities: i) a female may copulate indiscriminately to increase the likelihood of finding a genetically compatible male (Medsen et al., 1992), and ii) females may mate with more than one male to upgrade previous matings (Newport and Gromko, 1984). A number of possibilities have been suggested that reduce female survival including: i) mechanical damage or adverse physiological consequences due to mating or substances transfer during mating (Ikeda, 1974; Chapman et al., 1995; Lung et al., 2002), ii) parasite and disease transmission during copulation (Eberhard, 1996), iii) time and energy losses associated with mating (Ringo, 1996), and iv) increased vulnerability to predators while mating (Manning, 1962).
There is a conflict between female reproductive rate and male interests. Selection always favors males that induce females to make a large reproductive investment soon after copulation. But greater reproductive activity causes a decrease in lifespan and subsequent fecundity (Chapman et al., 1995; Rice, 1996). Male adaptations, such as mating plugs and polyspermy (Parker, 1970; Eberhard, 1996), also lower female fitness. Sgro and Partridge (1999) suggested that aging, mortality, and death rates evolved primarily because of damaging effects of reproduction earlier in life. Chapman et al. (1995) demonstrated that seminal fluid products (Acps) from the main cells of the male accessory gland are responsible for the cost of mating in females and that increased exposure to these products increases the female death rate. In their view this is true when a female remates with various males. Recently, Lung et al. (2002) reported that out of eight Drosophila seminal fluid proteins (Acps) and one non-Acp tested, only Acp26F was toxic when ectopically expressed. Further, they found that the toxicity to pre-adult male or female Drosophila occurs after one exposure, whereas multiple exposures are needed for toxicity to adult female flies. Of the Acp26F received by females during mating ~10% enters the circulatory system while ~90% remains in the reproductive tract. In the reproductive tract Acp26F goes to the lumen of the uterus and the female's sperm storage organs. Furthermore, they concluded that a small amount of this protein entering into the female's hemo-lymph could contribute to the cost of mating. However, many investigators suggested that even though mating is costly, it might still cost less than rejecting the male (Boorman and Parker, 1976). Imhoff et al. (1998) suggested that the high frequency of female remating observed in populations of D. melanogaster does not reduce the lifespan, as suggested by Chapman et al. (1995). Bundgaard and Barker (2000) reported that remating is costly and does not exist in D. buzzattii. The work of Singh and Singh (2001b,c) also supports the notion that remating is also not costly in D. ananassae. So it is clear from the different studies that this is still a controversial issue and more work is needed.
CONCLUSIONS
The present review on female remating, sperm competition and sexual selection in Drosophila shows that these phenomena occur in many species of Drosophila and are also found in many insects, nematodes, fishes, amphibians, reptiles, birds, and mammals, including human beings. Female remating is fundamental to evolutionary biology as it determines the pattern of sexual selection via sperm competition and sexual conflicts between partners. Female remating is a prerequisite for sperm competition, because the total impact of sperm competition on male fitness (Gromko and Pyle, 1978) and the significant effect of remating on female fitness (Pyle and Gromko, 1978) combine to make an excellent example of sexual selection. Sperm competition offers a unique opportunity to study adaptations shaped by the interacting forces of natural, sexual and antagonistic selection (Rice, 1996). The existence of sperm competition in Drosophila has been inferred from the proportion of progeny sired by the second male in double mating experiments. This approach has been used to quantify genetic variation underlying sperm competition, to elucidate the genetic basis, to identify the dependence of different male competitive abilities on the genotype of the females with which they mate and to discern the potential role of sperm competition in species isolation (Civetta, 1999).
The common outcome of sperm competition within most species is second male precedence. In Drosophila, females of many species mate with more than one male in nature, and in the laboratory the second male to mate generally fathers more than 90% of the offspring (Clark and Begun, 1998; Bundgaard and Barker, 2000; Singh and Singh, 2001b) although the mechanisms of sperm precedence in Drosophila remain unknown the phenomenon appears to be influenced in large part by the seminal fluid of the second male (Clark et al., 1995). Furthermore, variation among males in their fertilization success has been shown to be repeatable and heritable (Chapman et al., 1995; Clark et al., 1995; Hughes, 1997). The fertilization success of males can be determined by pre-mating factors such as ability to obtain copulation or by post-mating mechanisms such as the quantity of sperm they produce and transfer during mating, the success of their sperm in reaching and fertilizing an egg (Hunter and Birkhead, 2002), their ability to displace the sperm that females stored during previous matings (Civetta, 1999; Price et al., 1999), and their ability to prevent any other male from subsequently introducing sperm (mating plugs) or displacing their own sperm. Actually, the diversity in mechanisms affecting paternity testifies that the degree of competition among males for fertilization is a significant evolutionary force shaping species morphology and behavior (Danielsson, 1998).
There are direct benefits as well as genetic benefits to be gained by females by taking more than one mate. Whenever females mate more than once during a reproductive cycle and ejaculates overlap, sperm competition occurs (Parker, 1970). Males commonly try to prevent a female from remating by guarding her until she lays her eggs or by transferring factors in the ejaculate that switch off receptivity and stimulate oviposition. This reduces the risk of sperm competition for the mating male. Females, on the other hand, may benefit by remating sooner than is optimal for males. Therefore, along with the conflict over mating rate, there is often a conflict between the sexes over the timing of remating by females. In general sexual conflict can result in substantial costs to females, as males are selected to manipulate females to behave sub-optimally by investing the majority of their resources into the male's offspring even if it lowers their lifetime reproductive output (Rice, 1996).
It would be interesting and important to know both in mechanical and evolutionary terms, why first male sperm are more susceptible to incapacitation the longer they remain in storage. This phenomenon might explain how males avoid incapacitating the sperm in their own ejaculate despite their ability to displace and or incapacitate their own sperm once it is stored in a female (Gilchrist and Partridge, 1997). Females also play an important role in sperm incapacitation as they gradually alter the physiology of the sperm they store. Genetic differences may explain why female genotypes differ widely in the degree of last male precedence (Clark and Begun, 1998). Moreover, if females evolve differences in the way they alter stored sperm and males evolve ways to protect their sperm from alteration by particular females, this might explain the observation that a first male's success depends on both his genotype and that of his mate (Clark et al., 1999). Up to now, in Drosophila and many other species, research has involved the competition between males to have access to the females and the many male adaptations for sperm competition. However, some investigators also have advance the idea of female control over post-copulatory processes, for instance the cryptic female choice is so far restricted to a few insect species (Eberhard, 1996). It would be very important to know about the existence of cryptic female choice in different species of Drosophila and higher animals, and also to develop experimental designs to distinguish the difference between the male manipulation and cryptic female choice, which is really a difficult task for researchers.
There are many factors that affect the remating rate in females, such as amount of sperm stored, the components of male seminal fluid, the level of nutrition and egg laying, density, sexual maturity, body size, and different experimental designs (Gromko and Gerhart, 1984; Newport and Gromko, 1984; Letsinger and Gromko, 1985; Trevitt et al., 1988; Singh and Singh, 2001b). Female remating may be male driven, but female often directly solicit copulations from a number of males and it is becoming increasingly clear that females in a wide range of taxa are genetically polyandrous. Remating by females is an insurance against male sterility, increasing the genetic heterogeneity of the offspring and it is necessary for full fecundity and fertility (Richmond and Ehrman, 1974).
There is variation in genital morphology, sperm morphology and sperm storage organs (Pitnick et al., 1999). So it would also be interesting from an evolutionary point of view to know how sperm are transported and used by females in different species of Drosophila; this answers a major question, how the female reproductive organs are functioning. There are many studies that conclude that females benefit from remating in two ways, that is genetic and non-genetic, but it is still unclear whether this happens in a large number of species of Drosophila. It will also be important to know whether females of all species of Drosophila are willing to remate, because females of some species of Drosophila have been reported as monoandrous (D. nasuta; Ringo, 1996). We also need to know the role of duration of copulation in female remating and its impact on sperm precedence mechanisms. In many species there is shorter duration of copulation in the second mating in females, when compared to the first mating (Singh and Singh, 1999; Bundgaard and Barker, 2000) and in some species duration of copulation in the second mating is longer than for the first mating (Joly et al., 1991). It would be important from an evolutionary point of view to know whether this variation in duration of copulation is a female or male adaptation, or is characteristic of the species because it is reported that increased duration of copulation is used by males in Drosophila to guard a female against remating (Gilchrist and Partridge, 2000). However, according to Eberhard (1996) copulation duration may be a component of post-mating courtship, i.e., females may require some initial period of mating to evaluate the male before they permit the transfer of ejaculate.
Studies indicate a greater variation for sperm priority patterns in different species of Drosophila and it would be important to continue these studies both under laboratory and natural conditions to know how laboratory conditions mimic the natural situation in which reproductive strategies evolved not only at phenotypic level but also at a molecular level, in order to come up with important clues to the selective forces influencing sperm usage. Studies also suggested that there is cost of mating and remating when a female mates several times (Chapman et al., 1995). Recently, Lung et al. (2002) also indicated a cost associated with mating due to the seminal fluid protein Acp62F, present in the main cells of accessory glands of males. However, other papers (Imhoff et al., 1998; Bundgaard and Barker, 2000; Singh and Singh, 2001c) indicate a lack of cost associated with mating and remating. So there is still disagreement over results obtained by different workers on the cost of mating. It is important to continue the work on this subject in natural and laboratory populations of different species of Drosophila, using molecular methods to determine the role of male accessory gland proteins in sperm management, i.e., from storage to utilization in Drosophila, to know how many more types of proteins (Acp genes) are involved in the process, to know whether the molecule responsible for reduction in female life span is similar in all species, and whether there is inter-species variation for these Acp proteins.
Thus, from the different studies it is clear that females of different species remate frequently and remating frequency and remating time are heritable and controlled by polygenes.
ACKNOWLEDGMENTS
Financial assistance in the form of a Senior Research Fellowship from the Council of Scientific and Industrial Research (CSIR), New Delhi, to S.R. Singh is gratefully acknowledged. One of the authors (S.R.S.) also thanks Prof. Geoff A. Parker for his assistance in providing some important research papers in the field of sperm competition.
REFERENCES
Anderson, W.W. (1974). Frequent multiple insemination in a natural population of Drosophila pseudoobscura. Am. Nat. 108: 709-711.
Arnquist, G. and Nilson, T. (2000). The evolution of polyandry: Multiple mating and female fitness in insects. Anim. Behav. 60: 145-164.
Aspi, J. (1992). Incidence and adaptive significance of multiple mating in females of two boreal Drosophila virilis group species. Ann. Zool. Fenn. 29: 147-159.
Aspi, J. and Lankinen, P. (1992). Frequency of multiple insemination in a natural population of Drosophila montana. Hereditas 117: 169-177.
Baker, R.R. and Bellis, M.A. (1988). "Kamikaze" sperm in mammals? Anim. Behav. 36: 936-939.
Baker, R. and Bellis, M.A. (1993). Human sperm competition: ejaculate manipulation by females and a function for the female orgasm. Anim. Behav. 46: 887-909.
Barbadilla, A., Quezada-Diaz, J.E., Ruiz, A., Santos, M. and Fontedevila, A. (1991). The evolutionary history of Drosophila buzzatii. XVII. Double mating and sperm predominance. Genet. Sel. Evol. 23: 133-140.
Bateman, A.J. (1948). Intra-sexual selection in Drosophila. Heredity 2: 349-368.
Bernasconi, G. and Keller, L. (2001). Female polyandry affects their sons' reproductive success in the red flour beetle Tribolium castaneum. J. Evol. Biol. 14: 186-193.
Birkead, T.R. and Møller, A.P. (1998). Sperm Competition and Sexual Selection. Academic Press, London, England.
Boorman, E. and Parker, G.A. (1976). Sperm (ejaculate) competition in Drosophila melanogaster, and the reproductive value of females to males in relation to female age and mating status. Ecol. Entomol. 1: 145-155.
Bressec, C., Joly, D., Devaux, J. and Lachaise, D. (1991). Can we predict the mating pattern of Drosophila females from the sperm length distribution in males? Experientia 47: 111-114.
Bundgaard, J. and Barker, J.S.F. (2000). Remating, sperm transfer, and sperm displacement in cactophilic species Drosophila buzzatii Patterson & Wheeler (Diptera: Drosophilidae). Biol. J. Linn. Soc. 71: 145-164.
Chapman, T. and Partridge, L. (1996). Female fitness in Drosophila melanogaster: an interaction between the effect of nutrition and of encounter rate with males. Proc. R. Soc. London, B 263: 755-759.
Chapman, T., Trevitt, S. and Partridge, L. (1994). Remating and male derived nutrients in Drosophila melanogaster. J. Evol. Biol. 7: 51-69.
Chapman, T., Liddle, L.F., Kalb, J.M., Wolfner, M.F. and Partridge, L. (1995). Cost of mating in Drosophila melanogaster females is mediated by male accessory gland products. Nature 373: 241-244.
Civetta, A. (1999). Direct visualization of sperm competition and sperm storage in Drosophila. Curr. Biol. 9: 841-844.
Civetta, A. and Clark, A.G. (2000a). Chromosomal effects on male and female components of sperm precedence in Drosophila. Genet. Res. 75: 143-151.
Civetta, A. and Clark, A.G. (2000b). Correlated effects of sperm competition and postmating female mortality. Proc. Natl. Acad. Sci. USA 97: 13162-13165.
Civetta, A. and Singh, R.S. (1995). High divergence of reproductive tract proteins and their associates with postzygotic reproductive isolation in Drosophila melanogaster and D. virilis group species. J. Mol. Evol. 41: 1085-1095.
Clark, A.G. and Begun, D.J. (1998). Female genotypes affect sperm displacement in Drosophila. Genetics 149: 1487-1493.
Clark, A.G., Aguade, M., Prout, T., Harshman, L.G. and Langley, C.H. (1995). Variation in sperm displacement and its association with accessory gland protein loci in Drosophila melanogaster. Genetics 139: 189-201.
Clark, A.G., Begun, D.J. and Prout, T. (1999). Female x male interaction in Drosophila sperm competition. Science 283: 217-220.
Clark, A.G., Dermitzakis, E.T. and Civetta, A. (2000). Nontransitivity of sperm precedence in Drosophila. Evolution 54: 1030-1035.
Cobbs, G. (1977). Multiple insemination and male sexual selection in natural population of Drosophila pseudoobscura. Am. Nat. 111: 641-656.
Cook, P.A., Harvey, I.F. and Parker, G.A. (1997). Predicting variation in sperm precedence. Philos. Trans. R. Soc. Lond. B 352: 771-780.
Craddock, E.M. and Johnson, W.E. (1978). Multiple insemination in natural populations of Drosophila silvestris. Dros. Inf. Serv. 53: 138-139.
Curtsinger, J.W. (1991). Sperm competition and evolution of multiple mating. Am. Nat. 138: 93-102.
Danielsson, I. (1998). Mechanisms of sperm competition in insects. Ann. Zool. Fenn. 35: 241-257.
Darwin, C. (1859). On the Origin of Species by Means of Natural Selection. John Murray, London, England.
Darwin, C. (1871). The Descent of Man and Selection in Relation to Sex. John Murray, London, England.
Dobzhansky, Th. and Pavlovsky, O. (1967). Repeated mating and sperm mixing in Drosophila pseudoobscura. Am. Nat. 101: 527-533.
Eberhard, W.G. (1996). Female Control: Sexual Selection by Cryptic Female Choice. Princeton University Press, Princeton, NJ, USA.
Etges, W.J. and Heed, W.B. (1992). Remating effects on the genetic structure of female life histories in populations of Drosophila mojavensis. Heredity 68: 515-528.
Fukui, H.H. and Gromko, M.H. (1989). Female receptivity to remating and early fecundity in Drosophila melanogaster. Evolution 43: 1311-1315.
Fukui, H.H. and Gromko, M.H. (1991a). Genetic basis for remating in Drosophila melanogaster. IV. A chromosome substitution analysis. Behav. Genet. 21: 169-182.
Fukui, H.H. and Gromko, M.H. (1991b). Genetic basis for remating in Drosophila melanogaster. V. Biometrical and planned comparison analysis. Behav. Genet. 21: 183-197.
Fukui, H.H. and Gromko, M.H. (1991c). Genetic basis for remating in Drosophila melanogaster. VI. Recombination analysis. Behav. Genet. 21: 199-209.
Fuyama, Y. (1995). Genetic evidence that ovulation reduces sexual receptivity in Drosophila melanogaster females. Behav. Genet. 25: 581-587.
Garant, D., Dodson, J.J. and Bernatchez, L. (2001). A genetic evaluation of mating system and determinants of individual reproductive success in Atlantic Salmon (Salmo salar L.). J. Hered. 92: 132-145.
Gibson, R.M. and Jewell, P.A. (1982). Semen quality, female choice and multiple mating in domestic sheep: A test of Trivers' sexual competence hypothesis. Behaviour 90: 9-31.
Gilchrist, A.S. and Partridge, L. (1997). Heritability of pre-adult viability differences can explain apparent heritability of sperm displacement ability in Drosophila melanogaster. Proc. R. Soc. Lond. B 264: 1271-1275.
Gilchrist, A.S. and Partridge, L. (2000). Why it is difficult to model sperm displacement in Drosophila melanogaster: The relation between sperm transfer and copulation duration. Evolution 54: 534-542.
Greeff, J.M. and Parker, G.A. (2000). Spermicide by females: What should males do? Proc. R. Soc. Lond. 267: 1759-1763.
Griffiths, R.C., McKechnie, S.W. and McKenzie, J.A. (1982). Multiple mating and sperm displacement in a natural population of Drosophila melanogaster. Theor. Appl. Genet. 62: 89-96.
Gromko, M.H. and Gerhart, P.D. (1984). Increased density does not increase remating frequency in laboratory populations of Drosophila melanogaster. Evolution 38: 451-455.
Gromko, M.H. and Markow, T.A. (1993). Courtship and remating in field populations of Drosophila. Anim. Behav. 45: 253-262.
Gromko, M.H. and Newport, M.E.A. (1988a). Genetic basis for remating in Drosophila melanogaster. II. Response to selection based on the behaviour of one sex. Behav. Genet. 18: 621-632.
Gromko, M.H. and Newport, M.E.A. (1988b). Genetic basis for remating in Drosophila melanogaster. II. Correlated responses to selection for female remating speed. Behav. Genet. 18: 633-643.
Gromko, M.H. and Pyle, D.W. (1978). Sperm competition, male fitness, and repeated mating by female Drosophila melanogaster. Evolution 32: 588-593.
Gromko, M.H., Sheehan, K. and Richmond, R.C. (1980). Random mixing in two species of Drosophila. Am. Nat. 115: 467-479.
Gromko, M.H., Gilbert, D.G. and Richmond, R.C. (1984). Sperm transfer and use in the multiple mating system of Drosophila. In: Sperm Competition and the Evolution of Animal Mating Systems (Smith, R.L., ed.). Academic Press, New York, NY, USA, pp. 372-427.
Gwynne, D.T. (1984). Male mating effort, confidence of paternity and insect sperm competition. In: Sperm Competition and the Evolution of Animal Mating Systems (Smith, R.L., ed.). Academic Press, New York, NY, USA, pp. 117-150.
Haig, D. and Bergstrom, C.T. (1995). Multiple mating, sperm competition and meiotic drive. J. Evol. Biol. 8: 265-282.
Halliday, T. and Arnold, S.J. (1987). Multiple mating by females: a perspective from quantitative genetics. Anim. Behav. 35: 939-941.
Hardeland, R. (1972). Species differences in the diurnal rhythmicity of courtship behaviour within the melanogaster group of the genus Drosophila. Anim. Behav. 20: 170-174.
Harshman, L.G. and Clark, A.G. (1998). Interference of sperm competition from broods of field-caught Drosophila. Evolution 52: 1334-1341.
Harshman, L.G. and Prout, T. (1994). Sperm displacement without sperm transfer in Drosophila melanogaster. Evolution 48: 758-766.
Harshman, L.G., Hoffman, A.A. and Prout, T. (1988). Environmental effects on remating in Drosophila melanogaster. Evolution 42: 312-321.
Harvey, I.F. and Parker, G.A. (2000). 'Sloppy' sperm mixing and intra-specific variation in sperm precedence (P2) patterns. Proc. R. Soc. Lond. B 267: 2537-2542.
Heifetz, Y., Tram, U. and Wofner, M.F. (2001). Male contributions to egg production: the role of accessory gland products and sperm in Drosophila melanogaster. Proc. R. Soc. Lond. B 268: 175-180.
Hirai, Y. and Kimura, M.T. (1999). Effects of copulation duration on fertility and sexual receptivity of females of Drosophila elegans. Zool. Sci. 16: 417-421.
Hoenigsberg, H.F. (1960). Sexual behavior: a discussion. Evolution 14: 527-528.
Hoenigsberg, H.F. (1999). Female competition in mate choice decreases fitness in flies (Drosophila pseudoobscura) from Colombia. Genet. Mol. Biol. 22: 213-215.
Holland, B. and Rice, W.R. (1999). Experimental removal of sexual selection reverses inter-sexual antagonistc co-evolution and removes reproductive load. Proc. Natl. Acad. Sci. USA 96: 5083-5088.
Huck, W.W., Quinn, R.P. and Lisk, R.D. (1985). Determinants of mating success in the golden hamster (Mesocricetus auratus). Behav. Ecol. Sociobiol. 17: 239-252.
Hudak, M.J. and Gromko, M.H. (1989). Response to selection for early and late development of sexual maturity in Drosophila melanogaster. Anim. Behav. 38: 344-351.
Hughes, K.A. (1997). Quantitative genetics of sperm precedence in Drosophila melanogaster. Genetics 145: 139-151.
Hunter, F.M. and Birkhead, T.R. (2002). Sperm viability and sperm competition in insects. Curr. Biol. 12: 121-123.
Ikeda, H. (1974). Multiple copulation: An abnormal mating behaviour, which deleteriously affects fitness in Drosophila mercatorum. Mem. Ehime Univ. Nat. Sci. Ser. B 3: 18-28.
Imhoff, M., Harr, B., Brem, G. and Schlotterer, C. (1998). Multiple mating in wild Drosophila melanogaster revisited by microsatellite analysis. Mol. Ecol. 7: 915-917.
Joly, D. and Lachaise, D. (1993). Multiple mating frequency differs in two different geographic strains of Drosophila teissieri. Dros. Inf. Serv. 72: 126-127.
Joly, D., Cariou, M.L. and Lachaise, D. (1991). Can sperm competition explain sperm polymorphism in Drosophila teissieri? Evol. Biol. 5: 25-44.
Joly, D., Bressac, C. and Lachaise, D. (1995). Disentangling giant sperm. Nature 377: 202.
Keller, L. and Reeve, H.K. (1995). Why do females mate with multiple males? The sexually-selected sperm hypothesis. Adv. Stud. Behav. 24: 291-315.
LaMunyon, C.W. and Ward, S. (1999). Evolution of sperm size in nematodes: sperm competition favours larger sperm. Proc. R. Soc. Lond. B 266: 263-267.
Lefevre, G. and Jonsson, U.B. (1962). Sperm transfer, storage, displacement and utilization in Drosophila melanogaster. Genetics 47: 1719-1736.
Lessels, C.M. and Parker, G.A. (1999). Parent-offspring conflict: the full-sib-half-sib fallacy. Proc. R. Soc. Lond. B 266: 1637-1643.
Letsinger, J.T. and Gromko, M.H. (1985). The role of sperm numbers in sperm competition and female remating in Drosophila melanogaster. Genetics 66: 195-202.
Levine, L., Asmussen, M., Olvera, O., Powell, J.R., Delarosa, M.E., Salceda, V.M., Gaso, M.I., Gujman, J. and Anderson, W.W. (1980). Population genetics of Mexican Drosophila. V. A high rate of multiple insemination in a natural population of Drosophila pseudoobscura. Am. Nat. 116: 493-503.
Li, S.-H. and Brown, J.L. (2002). Reduction of maternal care: a new benefit of multiple mating? Behav. Ecol. 13: 87-93.
Loukas, M., Vergini, Y. and Krimbas, C.B. (1981). The genetics of Drosophila subobscura populations. XVII. Multiple insemination and sperm displacement in Drosophila suboscura. Genetica 57: 29-37.
Lung, O. and Wolfner, M.F. (2001). Identification and characterization of the major Drosophila melanogaster mating plug protein. Insect Biochem. Mol. Biol. 31: 543-551.
Lung, O., Tram, U., Finnerty, C.M., Eipper-Mains, M.A., Kalb, J.M. and Wolfner, M.F. (2002). The Drosophila melanogaster seminal fluid protein Acp62F is a protease inhibitor that is toxic upon ectopic expression. Genetics 160: 211-224.
Manning, A. (1962). A sperm factor affecting the receptivity of Drosophila melanogaster females. Nature 194: 252-253.
Markow, T.A. (1982). Mating systems of cactophilic Drosophila. In: Ecological Genetics and Evolution: The Cactus-Yeast-Drosophila Model System (Barker, J.R.F. and Starmer, W.T., eds.). Academic Press, Marrickville, Australia, pp. 273-287.
Markow, T.A. (1985). A comparative investigations of mating system of Drosophila hydei. Anim. Behav. 33: 775-781.
Markow, T.A. (2000). Forced matings in natural populations of Drosophila. Am. Nat. 156: 100-103.
Markow, T.A. and Ankey, P.F. (1984). Drosophila males contribute to oogenesis in a multiple mating species. Science 224: 302-303.
Markow, T.A., Gallagher, P.D. and Krebs, R.A. (1990). Ejaculate-derived nutritional contribution and female reproductive success in Drosophila mojavensis (Patterson and Low). Funct. Ecol. 4: 67-73.
Marks, R.W., Seager, R.D., Ayala, F.J. and Barr, L.G. (1988). Local ecology and multiple matings in a natural population of Drosophila melanogaster. Am. Nat. 131: 918-923.
Maynard-Smith, J. (1956). Fertility, mating behaviour and sexual selection in Drosophila subobscura. J. Genet. 54: 261-279.
McRobert, S.P., Adams, C.R., Wutjke, M., Frank, J. and Jackson, L.L. (1997). A comparison of female post-copulatory behaviour in Drosophila melanogaster and Drosophila biarmipes. J. Insect Behav. 10: 761-770.
Medsen, T., Shine, R., Loman, J. and Hakansson, T. (1992). Why do female adders copulate so frequently? Nature 355: 440-441.
Mery, F. and Joly, D. (2002). Multiple mating, sperm transfer and oviposition pattern in the giant species, Drosophila bifurca. J. Evol. Biol. 15: 49-56.
Milkman, R. and Zeitler, R.R. (1974). Concurrent multiple paternity in natural populations of Drosophila melanogaster. Genetics 78: 1191-1193.
Møller, A.P. (1994). Sexual Selection and the Barn Swallow. Oxford University Press, Oxford, England.
Neubaum, D.M. and Wolfner, M.F. (1999). Mated Drosophila melanogaster females require a seminal fluid protein, Acp 36PE to store sperm efficiently. Genetics 153: 845-857.
Newport, M.E.A. and Gromko, M.H. (1984). The effect of experimental design on female receptivity to remating and its impact on reproductive success in Drosophila melanogaster. Evolution 38: 1261-1272.
Nonidez, J.F. (1920). The internal phenomenon of reproduction in Drosophila. Biol. Bull. 39: 1261-1272.
Ochando, M.D., Reyes, A. and Ayala, F.J. (1996). Multiple paternity in two natural populations (Orchard and Vineyard) of Drosophila. Proc. Natl. Acad. Sci. USA 93: 11769-11773.
Parker, G.A. (1970). Sperm competition and its evolutionary consequences in the insects. Biol. Rev. 45: 525-568.
Parker, G.A. (1993). Sperm competition games: Sperm size and number under adult control. Proc. R. Soc. Lond. B 253: 245-254.
Parker, G.A. (1998). Sperm competition and the evolution of ejaculates: Toward a theory base. In: Sperm Competition and Sexual Selection (Birkhead, T.R. and Moller, A.P., eds.). Academic Press, New York, NY, USA, pp. 3-54.
Parker, G.A. and Simmons, L.W. (1991). A model of constant random sperm displacement during mating: Evidence from Scaptophaga. Proc. R. Soc. Lond. B 246: 107-115.
Parker, G.A., Simmons, L.W. and Kirk, H. (1990). Analysing sperm competition data: Simple models for predicting mechanisms. Behav. Ecol. Sociobiol. 27: 55-65.
Partridge, L. and Hurst, L.D. (1998). Sex and conflict. Science 281: 2003-2008.
Pearse, D.E. and Avise, J.C. (2001). Turtle mating systems: behavior, sperm storage and genetic paternity. J. Hered. 92: 206-211.
Pitnick, S. and Markow, T.A. (1994). Male gametic strategies: Sperm size, testes size and the allocation of ejaculate among successive mates by the sperm-limited fly Drosophila pachea and its relatives. Am. Nat. 143: 785-819.
Pitnick, S., Markow, T.A. and Riedly, M.F. (1991). Transfer of ejaculate and incorporation of male-derived substances by females in the nannoptera species group (Diptera: Drosophilidae). Evolution 45: 774-780.
Pitnick, S., Markow, T.A. and Spicer, G.S. (1995). Delayed male maturity is a cost of producing large sperm in Drosophila. Proc. Natl. Acad. Sci. USA 92: 10614-10618.
Pitnick, S., Markow, T. and Spicer, G.S. (1999). Evolution of multiple kinds of female sperm-storage organs in Drosophila. Evolution 53: 1804-1822.
Pitnick, S., Brown, W.D. and Miller, G.T. (2001). Evolution of female remating behaviour following experimental removal of sexual selection. Proc. R. Soc. Lond. 268: 557-563.
Polak, M., Wolf, L.L., Starmer, W.T. and Barker, J.S.F. (2001). Function of the mating plug in Drosophila hibisci Bock. Behav. Ecol. Sociobiol. 49: 196-205.
Price, C.S.C. (1997). Conspecific sperm precedence in Drosophila. Nature 388: 663-666.
Price, C.S.C., Dyer, K.A. and Coyne, J.A. (1999). Sperm competition between Drosophila males involves both displacement and incapacitation. Nature 400: 449-452.
Price, C.S.C., Kim, C.H., Posluszny, J. and Coyne, J.A. (2000). Mechanisms of conspecific sperm precedence. Evolution 54: 2028-2037.
Prout, T. and Bundgaard, J. (1977). The population genetics of sperm displacement. Genetics 85: 95-124.
Prout, T. and Clark, A.G. (1996). Polymorphism in genes that influences sperm displacement. Genetics 144: 401-408.
Pyle, D.W. and Gromko, M.H. (1978). Repeated mating by female Drosophila melanogaster: The adaptive importance. Experientia 34: 449-450.
Pyle, D.W. and Gromko, M.H. (1981). Genetic basis for repeated mating in Drosophila melanogaster. Am. Nat. 117: 133-146.
Reinhardt, K. (2000). Variation in sperm precedence in Chorthippus grasshoppers (Caelifera: Gomphocerinae). Physiol. Entomol. 25: 324-329.
Reynolds, J.D. (1996). Animal breeding systems. Trends Ecol. Evol. 11: 68-72.
Rice, R. (1996). Sexually antagonistic male adaptation triggered by experimental arrest of female evolution. Nature 381: 232-234.
Rice, W.R. and Holland, B. (1997). The enemies within: inter-genomic conflict, inter-locus contest evolution (ICE), and the intra-specific red queen. Behav. Ecol. Sociobiol. 41: 1-10.
Richmond, R.C. (1976). Frequency of multiple insemination in natural populations of Drosophila. Am. Nat. 110: 485-486.
Richmond, R.C. and Ehrman, L. (1974). The incidence of repeated mating in the superspecies Drosophila paulistorum. Experientia 30: 489-490.
Richmond, R.C. and Powell, J.R. (1970). Evidence of heterosis associated with an enzyme locus in a natural population. Proc. Natl. Acad. Sci. USA 67: 1264-1267.
Ridley, M. (1988). Mating frequency and fecundity in insects. Biol. Rev. 63: 509-549.
Ridley, M. (1989). The incidence of sperm displacement in insects: Four conjectures, one corroboration. Biol. J. Linn. Soc. 38: 349-367.
Ridley, M. (1993). Clutch size and mating frequency in parasitic Hymenoptera. Am. Nat. 142: 893-910.
Ringo, J. (1996). Sexual receptivity in insects. Ann. Rev. Entomol. 41: 473-494.
Schwartz, J.M. and Boake, C.R.B. (1992). Sexual dimorphism in remating in Hawaiian Drosophila species. Anim. Behav. 44: 231-238.
Scott, D. (1987). The timing of the sperm effect on female Drosophila melanogaster receptivity. Anim. Behav. 35: 142-149.
Service, P.M. and Vossbrink, R.E. (1996). Genetic variation in "First" male effect on egg laying and remating by female Drosophila melanogaster. Behav. Genet. 26: 39-48.
Sgro, C.M. and Partridge, L. (1999). A delayed wave of death from reproduction in Drosophila. Science 286: 2521-2524.
Sgro, C.M., Chapman, T. and Partridge, L. (1998). Sex-specific selection on time to remate in Drosophila melanogaster. Anim. Behav. 56: 1267-1278.
Simmons, L.W. and Parker, G.A. (1992). Individual variation in sperm competition success of yellow Dung flies, Scatophaga stercoraria. Evolution 46: 366-375.
Simmons, L.W. and Siva-Jothy, M.T. (1998). Sperm competition in insects: mechanisms and the potential for selection. In: Sperm Competition and Sexual Selection (Birkhead, T.R. and Moller, A.P., eds). Academic Press, San Diego, CA, USA, pp. 341-434.
Singh, B.N. (1996). Population and behaviour genetics of Drosophila ananassae. Genetica 97: 321-329.
Singh, B.N. (2000). Drosophila ananassae - a species characterised by several unusual genetic features. Curr. Sci. 78: 391-398.
Singh, B.N. and Singh, S.R. (1999). Female remating in Drosophila ananassae: Shorter duration of copulation during second mating as compared to first mating. J. Biosci. 24: 427-431.
Singh, S.R. and Singh, B.N. (2000). Male remating in Drosophila ananassae: Evidence for interstrain variation in remating time and shorter duration of copulation during second mating. Zool. Sci. 17: 389-393.
Singh, B.N. and Singh, S.R. (2001a). Female remating in Drosophila ananassae: Evidence for sperm displacement and greater productivity after remating. Zool. Sci. 18: 181-185.
Singh, S.R. and Singh, B.N. (2001b). Female remating in Drosophila ananassae: Evidence for the effect of density on female remating frequency. J. Insect Behav. 14: 659-668.
Singh, S.R. and Singh, B.N. (2001c). Female remating in Drosophila ananassae: Bidirectional selection for remating speed. Behav. Genet. 31: 361-370.
Smith, R.L. (1984). Sperm Competition and the Evolution of Animal Mating Systems. Academic Press, New York, NY, USA.
Snook, R. (1998). The risk of sperm competition and the evolution of sperm heteromorphism. Anim. Behav. 56: 1497-1507.
Snook, R. and So, Y.K. (2000). Associations between female remating behaviour, oogenesis and oviposition in Drosophila melanogaster and Drosophila pseudoobscura. J. Insect Physiol. 46: 1489-1496.
Snook, R.R. and Markow, T.A. (1996). Possible role of non-fertilizing sperm as a nutrient source for female Drosophila pseudoobscura (Diptera: Drosophilidae). Pan. Entomol. 72: 121-129.
Stalker, H.D. (1976). Enzymes and reproduction in natural populations of Drosophila euronotus. Genetics 84: 375-384.
Stamencovic-Radak, M., Partridge, L. and Andjelcovic, M. (1993). Genetic correlation between the sexes in Drosophila melanogaster: A reply to Butlin. Anim. Behav. 45: 405.
Stockley, P. (1999). Sperm selection and genetic incompatibility: does relatedness of mates affect male success in sperm competition? Proc. R. Soc. Lond. B 266: 1663-1669.
Tompkins, L. and Hall, J.C. (1983). Identification of brain sites controlling female receptivity in mosaics of Drosophila melanogaster. Genetics 103: 179-195.
Tompkins, L., Gross, A.C., Hall, J.C., Gailey, D.A. and Siegel, R.W. (1982). The role of female movement in the sexual behavior of Drosophila melanogaster. Behav. Genet. 12: 295-307.
Tram, U. and Wolfner, M.F. (1998). Seminal fluid regulation of female sexual attractiveness in Drosophila melanogaster. Proc. Nat. Acad. Sci. USA 95: 4051-4054.
Tram, U. and Wolfner, M.F. (1999). Male seminal fluid proteins are essential for sperm storage in Drosophila melanogaster. Genetics 153: 837-844.
Tregenza, T. and Wedell, N. (2000). Genetic compatibility, mate choice and patterns of parentage. Mol. Ecol. 9: 1013-1027.
Trevitt, S., Fowler, K. and Partridge, L. (1988). An effect of egg deposition on the subsequent fertility and remating frequency of female Drosophila melanogaster. J. Insect Physiol. 34: 821-828.
Turner, M.E. (1986). Multiple mating, sperm competition and the fertility component of fitness in Drosophila pseudoobscura. Fla. Entomol. 69: 121-128.
Turner, M.E. and Anderson, W.W. (1983). Multiple mating and female fitness in Drosophila pseudoobscura. Evolution 37: 714-723.
Turner, M.E. and Anderson, W.W. (1984). Sperm predominance among Drosophila pseudoobscura karyotypes. Evolution 38: 983-995.
van-Vianen, A. and Bijlsma, R. (1993). The adult component of selection in Drosophila melanogaster. Some aspects of early remating activity in females. Heredity 71: 269-276.
Walker, W.F. (1980). Sperm utilization strategies in non-social insects. Am. Nat. 115: 780-799.
Wolfner, M.F. (1997). Tokens of love: Functions and regulation of Drosophila male accessory gland products. Insect Biochem. Mol. Biol. 27: 179-192.
Wyckoff, G.J., Wang, W. and Wu C.-I. (2000). Rapid evolution of male reproductive genes in the descent of man. Nature 403: 304-309.
Yasui, Y. (1997). A "good-sperm" model can explain the evolution of multiple mating by females. Am. Nat. 149: 573-584.
Zahavi, A. (1977). The cost of honesty (further remarks on the handicap principle). J. Theor. Biol. 67: 603-605.
Zeh, J.E. and Zeh, D.W. (2001). Reproductive mode and the genetic benefits of polyandry. Anim. Behav. 61: 1051-1063.
|
|